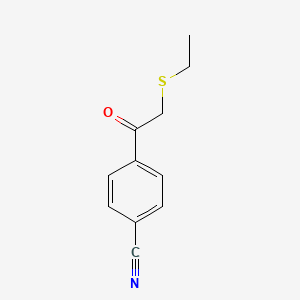
4-(2-(Ethylthio)acetyl)benzonitrile
- Click on QUICK INQUIRY to receive a quote from our team of experts.
- With the quality product at a COMPETITIVE price, you can focus more on your research.
Overview
Description
4-(2-(Ethylthio)acetyl)benzonitrile is an organic compound with the molecular formula C11H11NOS It is a derivative of benzonitrile, where the benzene ring is substituted with an ethylthioacetyl group at the para position
Preparation Methods
Synthetic Routes and Reaction Conditions
The synthesis of 4-(2-(Ethylthio)acetyl)benzonitrile typically involves the Friedel-Crafts acylation reaction. In this process, benzonitrile reacts with an ethylthioacetyl chloride in the presence of a Lewis acid catalyst such as aluminum chloride. The reaction is carried out under anhydrous conditions to prevent the hydrolysis of the acyl chloride. The general reaction scheme is as follows:
C6H5CN+CH3CH2SCOClAlCl3C6H4(COCH2CH2S)CN
Industrial Production Methods
Industrial production of this compound may involve similar synthetic routes but on a larger scale. The use of continuous flow reactors and optimized reaction conditions can enhance the yield and purity of the product. Additionally, green chemistry approaches, such as the use of ionic liquids as solvents and catalysts, can be employed to minimize environmental impact.
Chemical Reactions Analysis
Types of Reactions
4-(2-(Ethylthio)acetyl)benzonitrile can undergo various chemical reactions, including:
Oxidation: The ethylthio group can be oxidized to a sulfoxide or sulfone using oxidizing agents like hydrogen peroxide or m-chloroperbenzoic acid.
Reduction: The nitrile group can be reduced to an amine using reducing agents such as lithium aluminum hydride.
Substitution: The benzene ring can undergo electrophilic aromatic substitution reactions, such as nitration, sulfonation, and halogenation.
Common Reagents and Conditions
Oxidation: Hydrogen peroxide, m-chloroperbenzoic acid, acetic acid as solvent.
Reduction: Lithium aluminum hydride, tetrahydrofuran as solvent.
Substitution: Nitric acid for nitration, sulfuric acid for sulfonation, halogens (e.g., bromine) for halogenation.
Major Products Formed
Oxidation: Sulfoxides and sulfones.
Reduction: Primary amines.
Substitution: Nitro, sulfonic acid, and halogenated derivatives.
Scientific Research Applications
Scientific Research Applications
Organic Synthesis
4-(2-(Ethylthio)acetyl)benzonitrile is used as an intermediate in synthesizing complex organic molecules. It can undergo various reactions, demonstrating its versatility for further functionalization.
Biological Activity
Research indicates that this compound may have significant biological activity and has been explored for potential pharmacological properties, including antimicrobial and anticancer activities. The ethylthioacetyl group enhances its lipophilicity, which may facilitate interactions with lipid membranes and intracellular targets. This property is crucial for its effectiveness in biological systems, where it might interact with enzymes or receptors to modulate specific pathways.
Interaction Studies
Interaction studies involving this compound focus on its mechanism of action within biological systems. It may inhibit or activate specific biochemical pathways by interacting with various enzymes or receptors, and understanding these interactions is essential for developing its potential therapeutic applications.
Industrial Production
For industrial production, similar synthetic routes may be scaled up using continuous flow reactors and optimized conditions to enhance yield and purity. Green chemistry approaches, such as using ionic liquids as solvents and catalysts, are also being explored to minimize environmental impact.
Structural Considerations
Mechanism of Action
The mechanism of action of 4-(2-(Ethylthio)acetyl)benzonitrile depends on its specific application. In biological systems, it may interact with enzymes or receptors, leading to inhibition or activation of specific pathways. The ethylthioacetyl group can enhance the compound’s lipophilicity, facilitating its interaction with lipid membranes and intracellular targets.
Comparison with Similar Compounds
Similar Compounds
4-Acetylbenzonitrile: Similar structure but lacks the ethylthio group.
4-Formylbenzonitrile: Contains a formyl group instead of an acetyl group.
4-Hydroxybenzonitrile: Contains a hydroxyl group instead of an acetyl group.
Uniqueness
4-(2-(Ethylthio)acetyl)benzonitrile is unique due to the presence of the ethylthioacetyl group, which imparts distinct chemical and physical properties. This group can influence the compound’s reactivity, solubility, and biological activity, making it a valuable compound for various applications.
Biological Activity
4-(2-(Ethylthio)acetyl)benzonitrile is a compound that has garnered attention in pharmacological research due to its potential biological activities. This article aims to provide a comprehensive overview of its biological properties, focusing on its pharmacological effects, synthesis, structure-activity relationships (SAR), and relevant case studies.
Chemical Structure and Synthesis
This compound is characterized by the presence of an ethylthio group and a benzonitrile moiety. The compound can be synthesized through various organic reactions, including acylation and thiol substitution methods. The synthesis typically involves the reaction of benzonitrile derivatives with ethylthioacetyl chloride under basic conditions to yield the desired product.
Anticancer Properties
Research has shown that compounds featuring similar functional groups can exhibit anticancer activity. A study on 1,3,4-oxadiazol-2-yl derivatives indicated broad-spectrum anticancer activity with IC50 values in the low micromolar range . Given the structural similarities, it is plausible that this compound may also possess anticancer properties.
Structure-Activity Relationship (SAR)
The SAR studies of related compounds highlight the importance of substituents in modulating biological activity. For example, modifications on the phenyl ring significantly affect potency and selectivity against various cancer cell lines . In particular, electron-withdrawing groups tend to enhance activity, while bulky substituents may reduce it. This insight can guide further research into optimizing this compound for enhanced biological effects.
In Vivo Studies
In vivo evaluations using animal models have been pivotal in assessing the anticonvulsant potential of structurally related compounds. For instance, studies involving pentylenetetrazole-induced seizures revealed that certain derivatives provided significant protection against seizures, suggesting a mechanism involving GABA receptor modulation .
Comparative Analysis
A comparative analysis of related compounds demonstrates varying degrees of biological activity based on structural modifications. For example, compounds with thiomethyl substitutions retained substantial activity compared to their non-thiomethyl counterparts, indicating the significance of sulfur-containing groups in enhancing pharmacological effects .
Data Tables
Q & A
Q. What are the key synthetic routes for 4-(2-(Ethylthio)acetyl)benzonitrile, and how do reaction conditions influence yield?
Basic
The compound can be synthesized via nucleophilic substitution or coupling reactions. For example, lithiation of 3-bromothiophene with n-butyllithium at −78°C, followed by sulfur addition and reaction with 4-(2-bromoacetyl)benzonitrile, yields intermediates like 4-[2-(thiophen-3-ylsulfanyl)acetyl]benzonitrile . Ring closure using polyphosphoric acid (PPA) in refluxing chlorobenzene generates thienothiophene derivatives. Bromination with NBS at −10°C in DMF further modifies the structure . Yield optimization requires precise temperature control, inert atmospheres, and stoichiometric ratios of sulfur/alkylating agents.
Advanced
Advanced routes involve Suzuki-Miyaura coupling for polymer synthesis. For instance, 4-thieno[3,2-b]thiophen-3-ylbenzonitrile (TT-CN) was copolymerized with anthracene or biphenyl via Suzuki coupling, achieving molecular weights >20 kDa. Catalyst choice (e.g., Pd(PPh₃)₄ vs. Pd(OAc)₂) and solvent polarity (toluene vs. DMF) significantly impact regioselectivity and polydispersity indices (PDI ≤1.5) . Microwave-assisted methods may reduce reaction times but risk thermal decomposition of the nitrile group.
Q. How can spectroscopic techniques validate the structural integrity of this compound derivatives?
Basic
¹H NMR (500 MHz, DMSO-d₆) is critical for identifying key protons: aromatic protons (δ 8.15–8.17 ppm for benzonitrile), methylene groups (δ 4.84 ppm for CH₂), and thioether linkages (δ 2.5–3.0 ppm for S–CH₂). Mass spectrometry (EI, 70 eV) confirms molecular ions (e.g., m/z 277 [M+1] for a thiadiazol derivative) . Elemental analysis (C, H, N, S) should align with theoretical values within ±0.3% .
Advanced
High-resolution MALDI-TOF can resolve polymer end-groups (e.g., bromine-terminated vs. hydrogen-terminated chains) . X-ray crystallography (e.g., R factor ≤0.059) confirms bond angles and torsion angles in crystalline derivatives, revealing steric effects from substituents like trifluoromethyl groups . IR spectroscopy detects subtle changes in C≡N stretching frequencies (2220–2240 cm⁻¹) when electron-withdrawing groups are introduced .
Q. What are the applications of this compound in optoelectronic materials?
Basic
The compound serves as a precursor for thermally activated delayed fluorescence (TADF) emitters in OLEDs. Derivatives with phenoxazine-carbazole donors exhibit narrow singlet-triplet energy gaps (ΔEₛₜ ≤0.2 eV), enabling efficient blue emission .
Advanced
Copolymerization with anthracene enhances charge transport in hole-transport layers (HTLs). P1 (TT-biphenyl polymer) shows a hole mobility of 10⁻⁴ cm²/V·s, outperforming P3HT in device stability . Computational modeling (DFT, TD-DFT) predicts HOMO-LUMO distributions, guiding molecular design for external quantum efficiency (EQE) >12% in nondoped OLEDs .
Q. How do structural modifications of the benzonitrile core affect cytotoxicity in cancer research?
Advanced
Substitution at the acetyl position with triazole or phenoxy groups modulates apoptosis pathways. For example, 4-[2-(3-chlorophenyl)-1-(1H-1,2,4-triazol-1-yl)ethenyl]benzonitrile inhibits aromatase (IC₅₀ = 0.8 µM in MCF-7 cells), comparable to letrozole . SAR studies reveal that electron-withdrawing groups (e.g., -CF₃) enhance binding to cytochrome P450 enzymes, while bulky substituents reduce off-target effects .
Q. What are common pitfalls in analyzing conflicting data on reaction yields or biological activity?
Advanced
Contradictions often arise from impurities in intermediates (e.g., residual PPA in thienothiophene derivatives) or solvent polarity effects. For example, DMF increases bromination yields but may hydrolyze nitrile groups over time . In biological assays, discrepancies in IC₅₀ values (e.g., ±20% across labs) can stem from cell line heterogeneity (e.g., ER+ vs. ER− breast cancer models) . Statistical tools like ANOVA with post-hoc Tukey tests are recommended for cross-study comparisons.
Q. What methodologies are used to study degradation pathways or byproduct formation?
Advanced
Accelerated stability testing (40°C/75% RH for 6 months) identifies major degradation products via LC-MS. For example, oxidation of the ethylthio group generates sulfoxide derivatives, detectable at m/z +16 . Mechanistic studies using ¹⁸O-labeled H₂O or D₂O clarify hydrolysis pathways .
Q. How can researchers address challenges in crystallizing benzonitrile derivatives for structural analysis?
Advanced
Slow vapor diffusion (e.g., hexane/EtOAc) or seeding with isomorphic crystals improves success rates. For derivatives with flexible side chains (e.g., cyclohexyl groups), low-temperature crystallization (−20°C) reduces conformational disorder . Synchrotron X-ray sources enhance resolution for weakly diffracting crystals (<0.8 Å) .
Properties
CAS No. |
115505-06-1 |
---|---|
Molecular Formula |
C11H11NOS |
Molecular Weight |
205.28 g/mol |
IUPAC Name |
4-(2-ethylsulfanylacetyl)benzonitrile |
InChI |
InChI=1S/C11H11NOS/c1-2-14-8-11(13)10-5-3-9(7-12)4-6-10/h3-6H,2,8H2,1H3 |
InChI Key |
RIHYJARYAVCCMU-UHFFFAOYSA-N |
Canonical SMILES |
CCSCC(=O)C1=CC=C(C=C1)C#N |
Origin of Product |
United States |
Disclaimer and Information on In-Vitro Research Products
Please be aware that all articles and product information presented on BenchChem are intended solely for informational purposes. The products available for purchase on BenchChem are specifically designed for in-vitro studies, which are conducted outside of living organisms. In-vitro studies, derived from the Latin term "in glass," involve experiments performed in controlled laboratory settings using cells or tissues. It is important to note that these products are not categorized as medicines or drugs, and they have not received approval from the FDA for the prevention, treatment, or cure of any medical condition, ailment, or disease. We must emphasize that any form of bodily introduction of these products into humans or animals is strictly prohibited by law. It is essential to adhere to these guidelines to ensure compliance with legal and ethical standards in research and experimentation.