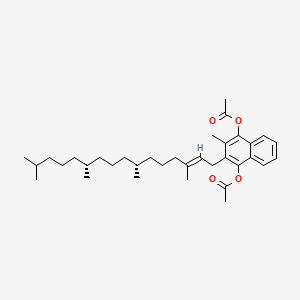
Vitamin K1 diacetate
Overview
Description
Vitamin K1 diacetate, also known as phylloquinone diacetate, is a synthetic derivative of Vitamin K1 (phylloquinone). Vitamin K1 is a fat-soluble vitamin that plays a crucial role in blood coagulation and bone metabolism. The diacetate form is often used in research and industrial applications due to its enhanced stability and solubility compared to its parent compound.
Mechanism of Action
Target of Action
Vitamin K1, also known as phylloquinone or phytonadione, is a fat-soluble vitamin . The primary targets of Vitamin K1 are the precursors to coagulation factors II, VII, IX, and X . These coagulation factors play a crucial role in the blood clotting process .
Mode of Action
Phylloquinone acts as a cofactor of the enzyme γ-carboxylase . This enzyme modifies and activates the precursors to the coagulation factors, thereby playing a significant role in the treatment of coagulation disorders due to faulty formation of these factors caused by deficiency or interference in the activity of vitamin K .
Biochemical Pathways
Vitamin K1 is essential for several physiological processes, such as blood coagulation . In this process, it serves as a cofactor for the conversion of peptide-bound glutamate to γ-carboxyglutamate in vitamin K-dependent proteins . This process is driven by the vitamin K cycle facilitated by γ-carboxyglutamyl carboxylase, vitamin K epoxide reductase, and ferroptosis suppressor protein-1 .
Pharmacokinetics
The pharmacokinetics of Vitamin K1 have been well documented . For instance, intravenous phylloquinone is 90% cleared in 2 hours, and 99% cleared in 8 hours . A 10 mg intravenous dose of phylloquinone has a mean clearance of 91 ± 24 mL/min . These properties impact the bioavailability of the compound and its therapeutic efficacy.
Result of Action
The primary result of Vitamin K1’s action is the prevention of hemorrhagic conditions in infants and the reversal of coumarin overdoses . By activating the coagulation factors, Vitamin K1 plays a crucial role in promoting normal blood clotting .
Action Environment
The action, efficacy, and stability of Vitamin K1 can be influenced by various environmental factors. For instance, Vitamin K1 is a fat-soluble vitamin , which means its absorption can be affected by the presence or absence of dietary fats. Furthermore, certain medications and health conditions can interfere with Vitamin K metabolism, thereby affecting its action .
Biochemical Analysis
Biochemical Properties
Vitamin K1 diacetate plays a crucial role in biochemical reactions, particularly in the carboxylation of specific proteins. This compound interacts with the enzyme gamma-glutamyl carboxylase, which catalyzes the carboxylation of glutamate residues in Vitamin K-dependent proteins. These proteins include coagulation factors II, VII, IX, and X, as well as proteins involved in bone metabolism, such as osteocalcin . The interaction between this compound and gamma-glutamyl carboxylase is essential for the activation of these proteins, enabling them to bind calcium ions and perform their biological functions.
Cellular Effects
This compound influences various cellular processes, including cell signaling pathways, gene expression, and cellular metabolism. In particular, this compound has been shown to affect the expression of genes involved in coagulation and bone metabolism . This compound also plays a role in the regulation of oxidative stress and inflammation, which are critical for maintaining cellular homeostasis. Additionally, this compound has been found to promote the differentiation of osteoblasts, the cells responsible for bone formation, and to inhibit the calcification of vascular smooth muscle cells .
Molecular Mechanism
The molecular mechanism of action of this compound involves its role as a cofactor for gamma-glutamyl carboxylase. This enzyme catalyzes the conversion of glutamate residues in Vitamin K-dependent proteins to gamma-carboxyglutamate residues, which are essential for calcium binding . The binding of this compound to gamma-glutamyl carboxylase enhances the enzyme’s activity, leading to the activation of coagulation factors and other proteins involved in bone metabolism. Additionally, this compound has been shown to modulate the activity of transcription factors, such as the steroid and xenobiotic receptor, which regulate the expression of genes involved in oxidative stress and inflammation .
Temporal Effects in Laboratory Settings
In laboratory settings, the effects of this compound can vary over time due to its stability and degradation. Studies have shown that this compound is relatively stable under controlled conditions, but it can degrade over time when exposed to light and air . The long-term effects of this compound on cellular function have been observed in both in vitro and in vivo studies. For example, prolonged exposure to this compound has been found to enhance the carboxylation of Vitamin K-dependent proteins and to promote bone formation in animal models .
Dosage Effects in Animal Models
The effects of this compound vary with different dosages in animal models. At low doses, this compound has been shown to enhance the carboxylation of coagulation factors and to improve bone health . At high doses, this compound can cause toxic effects, such as liver damage and impaired blood clotting . Threshold effects have also been observed, with certain dosages required to achieve optimal carboxylation of Vitamin K-dependent proteins and to promote bone formation .
Metabolic Pathways
This compound is involved in several metabolic pathways, including the Vitamin K cycle and the synthesis of gamma-carboxyglutamate residues . This compound interacts with enzymes such as gamma-glutamyl carboxylase and Vitamin K epoxide reductase, which are essential for the recycling of Vitamin K and the activation of Vitamin K-dependent proteins . Additionally, this compound has been found to influence metabolic flux and metabolite levels, particularly in pathways related to bone metabolism and coagulation .
Transport and Distribution
The transport and distribution of this compound within cells and tissues are influenced by its lipophilic nature. This compound is transported in the bloodstream by lipoproteins and is taken up by cells through receptor-mediated endocytosis . Within cells, this compound is distributed to various organelles, including the endoplasmic reticulum and mitochondria, where it exerts its biological effects . The interaction of this compound with transporters and binding proteins also affects its localization and accumulation in specific tissues .
Subcellular Localization
This compound is localized in several subcellular compartments, including the endoplasmic reticulum, mitochondria, and peroxisomes . The subcellular localization of this compound is essential for its activity, as it allows for the efficient carboxylation of Vitamin K-dependent proteins and the regulation of cellular processes such as oxidative stress and inflammation . Targeting signals and post-translational modifications, such as phosphorylation, direct this compound to specific compartments, ensuring its proper function within the cell .
Preparation Methods
Synthetic Routes and Reaction Conditions: The synthesis of Vitamin K1 diacetate typically involves the acetylation of Vitamin K1. The process begins with the extraction of Vitamin K1 from plant sources such as green leafy vegetables. The extracted Vitamin K1 is then subjected to acetylation using acetic anhydride in the presence of a catalyst such as pyridine. The reaction is carried out under controlled temperature and pressure conditions to ensure high yield and purity.
Industrial Production Methods: In industrial settings, the production of this compound follows a similar synthetic route but on a larger scale. The process involves the use of industrial-grade solvents and reagents, and the reaction conditions are optimized for mass production. The final product is purified using techniques such as crystallization and chromatography to achieve the desired quality.
Chemical Reactions Analysis
Types of Reactions: Vitamin K1 diacetate undergoes various chemical reactions, including:
Oxidation: The compound can be oxidized to form Vitamin K1 epoxide.
Reduction: It can be reduced back to its parent compound, Vitamin K1.
Substitution: The acetate groups can be substituted with other functional groups under specific conditions.
Common Reagents and Conditions:
Oxidation: Common oxidizing agents include hydrogen peroxide and potassium permanganate.
Reduction: Reducing agents such as sodium borohydride and lithium aluminum hydride are used.
Substitution: Reagents like sodium methoxide and other nucleophiles can be used for substitution reactions.
Major Products Formed:
Oxidation: Vitamin K1 epoxide.
Reduction: Vitamin K1.
Substitution: Various substituted derivatives depending on the nucleophile used.
Scientific Research Applications
Vitamin K1 diacetate has a wide range of applications in scientific research:
Chemistry: Used as a reagent in organic synthesis and as a standard in analytical chemistry.
Biology: Studied for its role in cellular processes and its effects on gene expression.
Medicine: Investigated for its potential therapeutic effects in conditions related to blood coagulation and bone health.
Industry: Used in the formulation of dietary supplements and fortified foods.
Comparison with Similar Compounds
Vitamin K1 diacetate is unique compared to other similar compounds due to its enhanced stability and solubility. Similar compounds include:
Vitamin K1 (Phylloquinone): The parent compound, less stable and less soluble.
Vitamin K2 (Menaquinone): A group of compounds with varying side chains, primarily involved in bone and cardiovascular health.
Vitamin K3 (Menadione): A synthetic form without the isoprenoid side chain, used in animal feed and some supplements.
This compound stands out due to its specific applications in research and industry, where stability and solubility are critical factors.
Properties
IUPAC Name |
[4-acetyloxy-2-methyl-3-[(E,7R,11R)-3,7,11,15-tetramethylhexadec-2-enyl]naphthalen-1-yl] acetate | |
---|---|---|
Source | PubChem | |
URL | https://pubchem.ncbi.nlm.nih.gov | |
Description | Data deposited in or computed by PubChem | |
InChI |
InChI=1S/C35H52O4/c1-24(2)14-11-15-25(3)16-12-17-26(4)18-13-19-27(5)22-23-31-28(6)34(38-29(7)36)32-20-9-10-21-33(32)35(31)39-30(8)37/h9-10,20-22,24-26H,11-19,23H2,1-8H3/b27-22+/t25-,26-/m1/s1 | |
Source | PubChem | |
URL | https://pubchem.ncbi.nlm.nih.gov | |
Description | Data deposited in or computed by PubChem | |
InChI Key |
OMMWEESMXJLLLQ-LGWQWDGZSA-N | |
Source | PubChem | |
URL | https://pubchem.ncbi.nlm.nih.gov | |
Description | Data deposited in or computed by PubChem | |
Canonical SMILES |
CC1=C(C2=CC=CC=C2C(=C1CC=C(C)CCCC(C)CCCC(C)CCCC(C)C)OC(=O)C)OC(=O)C | |
Source | PubChem | |
URL | https://pubchem.ncbi.nlm.nih.gov | |
Description | Data deposited in or computed by PubChem | |
Isomeric SMILES |
CC1=C(C2=CC=CC=C2C(=C1C/C=C(\C)/CCC[C@H](C)CCC[C@H](C)CCCC(C)C)OC(=O)C)OC(=O)C | |
Source | PubChem | |
URL | https://pubchem.ncbi.nlm.nih.gov | |
Description | Data deposited in or computed by PubChem | |
Molecular Formula |
C35H52O4 | |
Source | PubChem | |
URL | https://pubchem.ncbi.nlm.nih.gov | |
Description | Data deposited in or computed by PubChem | |
Molecular Weight |
536.8 g/mol | |
Source | PubChem | |
URL | https://pubchem.ncbi.nlm.nih.gov | |
Description | Data deposited in or computed by PubChem | |
CAS No. |
604-87-5 | |
Record name | Vitamin K1 diacetate | |
Source | ChemIDplus | |
URL | https://pubchem.ncbi.nlm.nih.gov/substance/?source=chemidplus&sourceid=0000604875 | |
Description | ChemIDplus is a free, web search system that provides access to the structure and nomenclature authority files used for the identification of chemical substances cited in National Library of Medicine (NLM) databases, including the TOXNET system. | |
Retrosynthesis Analysis
AI-Powered Synthesis Planning: Our tool employs the Template_relevance Pistachio, Template_relevance Bkms_metabolic, Template_relevance Pistachio_ringbreaker, Template_relevance Reaxys, Template_relevance Reaxys_biocatalysis model, leveraging a vast database of chemical reactions to predict feasible synthetic routes.
One-Step Synthesis Focus: Specifically designed for one-step synthesis, it provides concise and direct routes for your target compounds, streamlining the synthesis process.
Accurate Predictions: Utilizing the extensive PISTACHIO, BKMS_METABOLIC, PISTACHIO_RINGBREAKER, REAXYS, REAXYS_BIOCATALYSIS database, our tool offers high-accuracy predictions, reflecting the latest in chemical research and data.
Strategy Settings
Precursor scoring | Relevance Heuristic |
---|---|
Min. plausibility | 0.01 |
Model | Template_relevance |
Template Set | Pistachio/Bkms_metabolic/Pistachio_ringbreaker/Reaxys/Reaxys_biocatalysis |
Top-N result to add to graph | 6 |
Feasible Synthetic Routes
Disclaimer and Information on In-Vitro Research Products
Please be aware that all articles and product information presented on BenchChem are intended solely for informational purposes. The products available for purchase on BenchChem are specifically designed for in-vitro studies, which are conducted outside of living organisms. In-vitro studies, derived from the Latin term "in glass," involve experiments performed in controlled laboratory settings using cells or tissues. It is important to note that these products are not categorized as medicines or drugs, and they have not received approval from the FDA for the prevention, treatment, or cure of any medical condition, ailment, or disease. We must emphasize that any form of bodily introduction of these products into humans or animals is strictly prohibited by law. It is essential to adhere to these guidelines to ensure compliance with legal and ethical standards in research and experimentation.