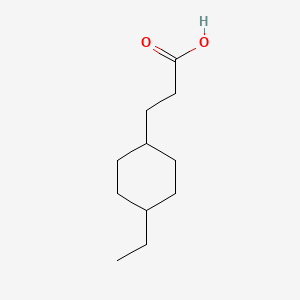
3-((1r,4r)-4-Ethylcyclohexyl)propanoic acid
- Click on QUICK INQUIRY to receive a quote from our team of experts.
- With the quality product at a COMPETITIVE price, you can focus more on your research.
Overview
Description
3-((1r,4r)-4-Ethylcyclohexyl)propanoic acid is an organic compound characterized by a cyclohexane ring substituted with an ethyl group and a propanoic acid moiety
Preparation Methods
Synthetic Routes and Reaction Conditions
The synthesis of 3-((1r,4r)-4-Ethylcyclohexyl)propanoic acid typically involves the following steps:
Cyclohexane Derivatization: Starting with cyclohexane, an ethyl group is introduced at the 4-position through a Friedel-Crafts alkylation reaction using ethyl chloride and aluminum chloride as a catalyst.
Hydrogenation: The resulting ethylcyclohexane is subjected to hydrogenation to ensure the correct stereochemistry (1r,4r).
Carboxylation: The ethylcyclohexane derivative is then carboxylated using a Grignard reagent, such as ethylmagnesium bromide, followed by carbonation with carbon dioxide to introduce the propanoic acid group.
Industrial Production Methods
Industrial production of this compound may involve similar steps but on a larger scale, with optimizations for yield and cost-effectiveness. Continuous flow reactors and advanced catalytic systems are often employed to enhance efficiency and scalability.
Chemical Reactions Analysis
Types of Reactions
Oxidation: This compound can undergo oxidation reactions, typically using reagents like potassium permanganate or chromium trioxide, to form corresponding ketones or carboxylic acids.
Reduction: Reduction reactions can be performed using hydrogen gas in the presence of a palladium catalyst to reduce any unsaturated bonds.
Substitution: Nucleophilic substitution reactions can occur at the propanoic acid moiety, where the carboxyl group can be replaced by other functional groups using reagents like thionyl chloride.
Common Reagents and Conditions
Oxidation: Potassium permanganate (KMnO₄), chromium trioxide (CrO₃)
Reduction: Hydrogen gas (H₂) with palladium (Pd) catalyst
Substitution: Thionyl chloride (SOCl₂)
Major Products
Oxidation: Ketones, carboxylic acids
Reduction: Saturated hydrocarbons
Substitution: Various substituted derivatives depending on the nucleophile used
Scientific Research Applications
Chemistry
In chemistry, 3-((1r,4r)-4-Ethylcyclohexyl)propanoic acid is used as a building block for the synthesis of more complex molecules. Its unique structure allows for the exploration of stereochemical effects in various reactions.
Biology
In biological research, this compound can be used to study the effects of cyclohexane derivatives on biological systems. It may serve as a model compound for understanding the metabolism and biotransformation of similar structures.
Medicine
Medically, derivatives of this compound are investigated for their potential therapeutic properties, including anti-inflammatory and analgesic effects. The compound’s structure-activity relationship (SAR) is of particular interest in drug design.
Industry
In the industrial sector, this compound can be used in the production of specialty chemicals and materials. Its stability and reactivity make it suitable for various applications, including polymer synthesis and as an intermediate in organic synthesis.
Mechanism of Action
The mechanism of action of 3-((1r,4r)-4-Ethylcyclohexyl)propanoic acid involves its interaction with specific molecular targets, such as enzymes or receptors. The propanoic acid moiety can participate in hydrogen bonding and electrostatic interactions, while the cyclohexane ring provides hydrophobic interactions. These interactions can modulate the activity of biological targets, leading to various physiological effects.
Comparison with Similar Compounds
Similar Compounds
Cyclohexylpropanoic acid: Lacks the ethyl substitution, leading to different steric and electronic properties.
4-Ethylcyclohexanecarboxylic acid: Similar structure but with the carboxyl group directly attached to the cyclohexane ring.
3-((1r,4r)-4-Methylcyclohexyl)propanoic acid: Similar structure but with a methyl group instead of an ethyl group.
Uniqueness
3-((1r,4r)-4-Ethylcyclohexyl)propanoic acid is unique due to the specific placement of the ethyl group and the propanoic acid moiety, which confer distinct steric and electronic characteristics. These features can influence its reactivity and interactions with biological targets, making it a valuable compound for research and industrial applications.
Properties
IUPAC Name |
3-(4-ethylcyclohexyl)propanoic acid |
Source
|
---|---|---|
Details | Computed by Lexichem TK 2.7.0 (PubChem release 2021.10.14) | |
Source | PubChem | |
URL | https://pubchem.ncbi.nlm.nih.gov | |
Description | Data deposited in or computed by PubChem | |
InChI |
InChI=1S/C11H20O2/c1-2-9-3-5-10(6-4-9)7-8-11(12)13/h9-10H,2-8H2,1H3,(H,12,13) |
Source
|
Details | Computed by InChI 1.0.6 (PubChem release 2021.10.14) | |
Source | PubChem | |
URL | https://pubchem.ncbi.nlm.nih.gov | |
Description | Data deposited in or computed by PubChem | |
InChI Key |
PKZPCVLZVDCNKZ-UHFFFAOYSA-N |
Source
|
Details | Computed by InChI 1.0.6 (PubChem release 2021.10.14) | |
Source | PubChem | |
URL | https://pubchem.ncbi.nlm.nih.gov | |
Description | Data deposited in or computed by PubChem | |
Canonical SMILES |
CCC1CCC(CC1)CCC(=O)O |
Source
|
Details | Computed by OEChem 2.3.0 (PubChem release 2021.10.14) | |
Source | PubChem | |
URL | https://pubchem.ncbi.nlm.nih.gov | |
Description | Data deposited in or computed by PubChem | |
Molecular Formula |
C11H20O2 |
Source
|
Details | Computed by PubChem 2.2 (PubChem release 2021.10.14) | |
Source | PubChem | |
URL | https://pubchem.ncbi.nlm.nih.gov | |
Description | Data deposited in or computed by PubChem | |
Molecular Weight |
184.27 g/mol |
Source
|
Details | Computed by PubChem 2.2 (PubChem release 2021.10.14) | |
Source | PubChem | |
URL | https://pubchem.ncbi.nlm.nih.gov | |
Description | Data deposited in or computed by PubChem | |
Disclaimer and Information on In-Vitro Research Products
Please be aware that all articles and product information presented on BenchChem are intended solely for informational purposes. The products available for purchase on BenchChem are specifically designed for in-vitro studies, which are conducted outside of living organisms. In-vitro studies, derived from the Latin term "in glass," involve experiments performed in controlled laboratory settings using cells or tissues. It is important to note that these products are not categorized as medicines or drugs, and they have not received approval from the FDA for the prevention, treatment, or cure of any medical condition, ailment, or disease. We must emphasize that any form of bodily introduction of these products into humans or animals is strictly prohibited by law. It is essential to adhere to these guidelines to ensure compliance with legal and ethical standards in research and experimentation.