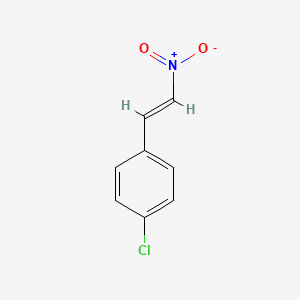
1-(4-Chlorophenyl)-2-nitroethene
Overview
Description
1-(4-Chlorophenyl)-2-nitroethene is an organic compound characterized by the presence of a nitro group and a chlorophenyl group attached to an ethene backbone
Preparation Methods
Synthetic Routes and Reaction Conditions: 1-(4-Chlorophenyl)-2-nitroethene can be synthesized through several methods. One common approach involves the nitration of 4-chlorostyrene using a nitrating agent such as nitric acid in the presence of a catalyst. The reaction typically occurs under controlled temperature conditions to ensure the selective formation of the nitroethene derivative.
Industrial Production Methods: Industrial production of this compound may involve large-scale nitration processes using continuous flow reactors. These reactors allow for precise control over reaction parameters, ensuring high yield and purity of the final product. The use of advanced purification techniques, such as recrystallization and chromatography, further enhances the quality of the compound.
Chemical Reactions Analysis
Types of Reactions: 1-(4-Chlorophenyl)-2-nitroethene undergoes various chemical reactions, including:
Reduction: The nitro group can be reduced to an amino group using reducing agents like tin(II) chloride or hydrogen in the presence of a catalyst.
Substitution: The chlorophenyl group can participate in nucleophilic substitution reactions, where the chlorine atom is replaced by other nucleophiles.
Oxidation: The ethene backbone can be oxidized to form epoxides or other oxygenated derivatives.
Common Reagents and Conditions:
Reduction: Tin(II) chloride in hydrochloric acid or catalytic hydrogenation.
Substitution: Nucleophiles such as amines or thiols in the presence of a base.
Oxidation: Oxidizing agents like potassium permanganate or m-chloroperbenzoic acid.
Major Products Formed:
Reduction: 1-(4-Chlorophenyl)-2-aminoethene.
Substitution: Various substituted derivatives depending on the nucleophile used.
Oxidation: Epoxides or hydroxylated derivatives.
Scientific Research Applications
1-(4-Chlorophenyl)-2-nitroethene has several applications in scientific research:
Chemistry: It serves as a building block for the synthesis of more complex organic molecules. Its reactivity makes it useful in developing new synthetic methodologies.
Biology: The compound can be used in studies involving enzyme inhibition and receptor binding due to its structural similarity to biologically active molecules.
Medicine: Research into its potential as a precursor for pharmaceutical compounds is ongoing, particularly in the development of anti-inflammatory and anticancer agents.
Industry: It is used in the production of specialty chemicals and intermediates for various industrial applications.
Mechanism of Action
The mechanism of action of 1-(4-Chlorophenyl)-2-nitroethene involves its interaction with molecular targets through its nitro and chlorophenyl groups. The nitro group can undergo reduction to form reactive intermediates that interact with biological macromolecules, potentially leading to enzyme inhibition or receptor modulation. The chlorophenyl group can enhance the compound’s binding affinity to specific targets, contributing to its biological activity.
Comparison with Similar Compounds
1-(4-Bromophenyl)-2-nitroethene: Similar structure but with a bromine atom instead of chlorine, leading to different reactivity and biological activity.
1-(4-Fluorophenyl)-2-nitroethene: Contains a fluorine atom, which can affect its electronic properties and interactions with biological targets.
1-(4-Methylphenyl)-2-nitroethene: The presence of a methyl group can influence the compound’s hydrophobicity and metabolic stability.
Uniqueness: 1-(4-Chlorophenyl)-2-nitroethene is unique due to the specific electronic and steric effects imparted by the chlorine atom. These effects can influence the compound’s reactivity and interactions with biological targets, making it a valuable compound for research and industrial applications.
Properties
IUPAC Name |
1-chloro-4-[(E)-2-nitroethenyl]benzene | |
---|---|---|
Source | PubChem | |
URL | https://pubchem.ncbi.nlm.nih.gov | |
Description | Data deposited in or computed by PubChem | |
InChI |
InChI=1S/C8H6ClNO2/c9-8-3-1-7(2-4-8)5-6-10(11)12/h1-6H/b6-5+ | |
Source | PubChem | |
URL | https://pubchem.ncbi.nlm.nih.gov | |
Description | Data deposited in or computed by PubChem | |
InChI Key |
GLJATYFHELDGEA-AATRIKPKSA-N | |
Source | PubChem | |
URL | https://pubchem.ncbi.nlm.nih.gov | |
Description | Data deposited in or computed by PubChem | |
Canonical SMILES |
C1=CC(=CC=C1C=C[N+](=O)[O-])Cl | |
Source | PubChem | |
URL | https://pubchem.ncbi.nlm.nih.gov | |
Description | Data deposited in or computed by PubChem | |
Isomeric SMILES |
C1=CC(=CC=C1/C=C/[N+](=O)[O-])Cl | |
Source | PubChem | |
URL | https://pubchem.ncbi.nlm.nih.gov | |
Description | Data deposited in or computed by PubChem | |
Molecular Formula |
C8H6ClNO2 | |
Source | PubChem | |
URL | https://pubchem.ncbi.nlm.nih.gov | |
Description | Data deposited in or computed by PubChem | |
DSSTOX Substance ID |
DTXSID30879307 | |
Record name | 4-CHLORO B-NITROSTYRENE | |
Source | EPA DSSTox | |
URL | https://comptox.epa.gov/dashboard/DTXSID30879307 | |
Description | DSSTox provides a high quality public chemistry resource for supporting improved predictive toxicology. | |
Molecular Weight |
183.59 g/mol | |
Source | PubChem | |
URL | https://pubchem.ncbi.nlm.nih.gov | |
Description | Data deposited in or computed by PubChem | |
CAS No. |
706-07-0, 5153-70-8 | |
Record name | Styrene, p-chloro-beta-nitro- | |
Source | ChemIDplus | |
URL | https://pubchem.ncbi.nlm.nih.gov/substance/?source=chemidplus&sourceid=0000706070 | |
Description | ChemIDplus is a free, web search system that provides access to the structure and nomenclature authority files used for the identification of chemical substances cited in National Library of Medicine (NLM) databases, including the TOXNET system. | |
Record name | 706-07-0 | |
Source | DTP/NCI | |
URL | https://dtp.cancer.gov/dtpstandard/servlet/dwindex?searchtype=NSC&outputformat=html&searchlist=2547 | |
Description | The NCI Development Therapeutics Program (DTP) provides services and resources to the academic and private-sector research communities worldwide to facilitate the discovery and development of new cancer therapeutic agents. | |
Explanation | Unless otherwise indicated, all text within NCI products is free of copyright and may be reused without our permission. Credit the National Cancer Institute as the source. | |
Record name | 4-CHLORO B-NITROSTYRENE | |
Source | EPA DSSTox | |
URL | https://comptox.epa.gov/dashboard/DTXSID30879307 | |
Description | DSSTox provides a high quality public chemistry resource for supporting improved predictive toxicology. | |
Record name | trans-4-Chloro-β-nitrostyrene | |
Source | European Chemicals Agency (ECHA) | |
URL | https://echa.europa.eu/information-on-chemicals | |
Description | The European Chemicals Agency (ECHA) is an agency of the European Union which is the driving force among regulatory authorities in implementing the EU's groundbreaking chemicals legislation for the benefit of human health and the environment as well as for innovation and competitiveness. | |
Explanation | Use of the information, documents and data from the ECHA website is subject to the terms and conditions of this Legal Notice, and subject to other binding limitations provided for under applicable law, the information, documents and data made available on the ECHA website may be reproduced, distributed and/or used, totally or in part, for non-commercial purposes provided that ECHA is acknowledged as the source: "Source: European Chemicals Agency, http://echa.europa.eu/". Such acknowledgement must be included in each copy of the material. ECHA permits and encourages organisations and individuals to create links to the ECHA website under the following cumulative conditions: Links can only be made to webpages that provide a link to the Legal Notice page. | |
Record name | 5153-70-8 | |
Source | European Chemicals Agency (ECHA) | |
URL | https://echa.europa.eu/information-on-chemicals | |
Description | The European Chemicals Agency (ECHA) is an agency of the European Union which is the driving force among regulatory authorities in implementing the EU's groundbreaking chemicals legislation for the benefit of human health and the environment as well as for innovation and competitiveness. | |
Explanation | Use of the information, documents and data from the ECHA website is subject to the terms and conditions of this Legal Notice, and subject to other binding limitations provided for under applicable law, the information, documents and data made available on the ECHA website may be reproduced, distributed and/or used, totally or in part, for non-commercial purposes provided that ECHA is acknowledged as the source: "Source: European Chemicals Agency, http://echa.europa.eu/". Such acknowledgement must be included in each copy of the material. ECHA permits and encourages organisations and individuals to create links to the ECHA website under the following cumulative conditions: Links can only be made to webpages that provide a link to the Legal Notice page. | |
Synthesis routes and methods I
Procedure details
Synthesis routes and methods II
Procedure details
Retrosynthesis Analysis
AI-Powered Synthesis Planning: Our tool employs the Template_relevance Pistachio, Template_relevance Bkms_metabolic, Template_relevance Pistachio_ringbreaker, Template_relevance Reaxys, Template_relevance Reaxys_biocatalysis model, leveraging a vast database of chemical reactions to predict feasible synthetic routes.
One-Step Synthesis Focus: Specifically designed for one-step synthesis, it provides concise and direct routes for your target compounds, streamlining the synthesis process.
Accurate Predictions: Utilizing the extensive PISTACHIO, BKMS_METABOLIC, PISTACHIO_RINGBREAKER, REAXYS, REAXYS_BIOCATALYSIS database, our tool offers high-accuracy predictions, reflecting the latest in chemical research and data.
Strategy Settings
Precursor scoring | Relevance Heuristic |
---|---|
Min. plausibility | 0.01 |
Model | Template_relevance |
Template Set | Pistachio/Bkms_metabolic/Pistachio_ringbreaker/Reaxys/Reaxys_biocatalysis |
Top-N result to add to graph | 6 |
Feasible Synthetic Routes
Disclaimer and Information on In-Vitro Research Products
Please be aware that all articles and product information presented on BenchChem are intended solely for informational purposes. The products available for purchase on BenchChem are specifically designed for in-vitro studies, which are conducted outside of living organisms. In-vitro studies, derived from the Latin term "in glass," involve experiments performed in controlled laboratory settings using cells or tissues. It is important to note that these products are not categorized as medicines or drugs, and they have not received approval from the FDA for the prevention, treatment, or cure of any medical condition, ailment, or disease. We must emphasize that any form of bodily introduction of these products into humans or animals is strictly prohibited by law. It is essential to adhere to these guidelines to ensure compliance with legal and ethical standards in research and experimentation.