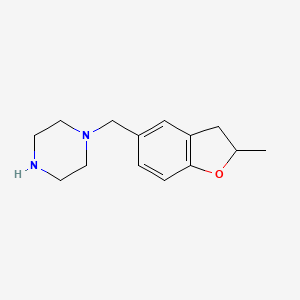
1-((2-Methyl-2,3-dihydrobenzofuran-5-yl)methyl)piperazine
- Click on QUICK INQUIRY to receive a quote from our team of experts.
- With the quality product at a COMPETITIVE price, you can focus more on your research.
Overview
Description
1-((2-Methyl-2,3-dihydrobenzofuran-5-yl)methyl)piperazine is a chemical compound with the molecular formula C14H20N2O. It is a derivative of benzofuran and piperazine, combining the structural features of both these compounds. Benzofuran derivatives are known for their diverse biological activities, making them significant in medicinal chemistry .
Preparation Methods
The synthesis of 1-((2-Methyl-2,3-dihydrobenzofuran-5-yl)methyl)piperazine typically involves the following steps:
Starting Materials: The synthesis begins with commercially available 2-allylphenol.
Iodocyclization: This step involves the iodocyclization of 2-allylphenol to form the benzofuran ring.
Industrial production methods may involve similar steps but are optimized for large-scale synthesis, ensuring high yield and purity.
Chemical Reactions Analysis
1-((2-Methyl-2,3-dihydrobenzofuran-5-yl)methyl)piperazine undergoes various chemical reactions, including:
Oxidation: The compound can be oxidized using common oxidizing agents like potassium permanganate or chromium trioxide.
Reduction: Reduction reactions can be carried out using agents like lithium aluminum hydride.
Substitution: The compound can undergo nucleophilic substitution reactions, particularly at the piperazine ring.
Common reagents and conditions used in these reactions include dichloromethane, methanol, and silica gel for purification . Major products formed from these reactions depend on the specific conditions and reagents used.
Scientific Research Applications
1-((2-Methyl-2,3-dihydrobenzofuran-5-yl)methyl)piperazine has several scientific research applications:
Chemistry: It is used as an intermediate in the synthesis of more complex organic molecules.
Mechanism of Action
The mechanism of action of 1-((2-Methyl-2,3-dihydrobenzofuran-5-yl)methyl)piperazine involves its interaction with specific molecular targets and pathways. Benzofuran derivatives are known to exhibit their effects through various mechanisms, including inhibition of enzymes, interaction with DNA, and modulation of cellular signaling pathways . The exact molecular targets and pathways involved depend on the specific biological activity being studied.
Comparison with Similar Compounds
1-((2-Methyl-2,3-dihydrobenzofuran-5-yl)methyl)piperazine can be compared with other benzofuran derivatives, such as:
2-Methyl-2,3-dihydrobenzofuran: This compound shares the benzofuran core but lacks the piperazine moiety.
Psoralen: A benzofuran derivative used in the treatment of skin diseases like psoriasis.
8-Methoxypsoralen: Another benzofuran derivative with similar applications as psoralen.
The uniqueness of this compound lies in its combined structural features of benzofuran and piperazine, which may confer distinct biological activities and applications.
Biological Activity
1-((2-Methyl-2,3-dihydrobenzofuran-5-yl)methyl)piperazine is a compound of significant interest in medicinal chemistry due to its potential biological activities. This article explores its interactions, pharmacological properties, and implications in therapeutic applications.
Chemical Structure and Properties
The molecular formula of this compound is C₁₃H₁₈N₂O, which indicates the presence of carbon, hydrogen, nitrogen, and oxygen. The compound features a piperazine ring linked to a substituted benzofuran moiety, contributing to its unique biological profile.
Binding Affinity to Histamine Receptors
Research has primarily focused on the binding affinity of this compound to histamine receptors, particularly H3 and H4. These receptors are implicated in various conditions such as inflammation, asthma, and neurodegenerative diseases like Alzheimer's and Parkinson's. Studies have shown that derivatives of this compound exhibit significant pharmacological activities by targeting these receptors effectively .
Table 1: Binding Affinities of Related Compounds
Compound Name | Histamine Receptor Type | Binding Affinity (Kd) |
---|---|---|
LINS01003 | H3 | 185 µM |
LINS01004 | H4 | 368 µM |
LINS01011 | H3 | Not determined |
LINS01018 | H4 | Not determined |
Neurotoxicity Assessment
A recent study evaluated the neurotoxicity of several synthetic derivatives of this compound (LINS01 series). The assessment utilized SH-SY5Y cell line cultures to measure cell viability through various assays (MTT, LDH). The results indicated that most compounds did not exhibit neurotoxic effects at concentrations up to 10 µM. Notably, LINS01011 significantly reduced lactate dehydrogenase (LDH) release in the presence of cocaine-induced neurotoxicity, suggesting a protective role against dopamine-related neurotoxicity .
Anti-inflammatory Properties
Another study highlighted the anti-inflammatory potential of piperazine derivatives similar to this compound. These compounds were evaluated for their efficacy in reducing inflammation through their action on histamine receptors. The findings demonstrated that modifications on the benzofuran ring significantly influenced receptor selectivity and potency .
Structure-Activity Relationship (SAR)
The structure-activity relationship plays a crucial role in understanding how modifications to the benzofuran moiety affect biological activity. For instance, variations in substitution patterns have been shown to alter binding affinities and receptor selectivity significantly. This insight is vital for designing more effective analogs with improved therapeutic profiles.
Table 2: Structural Variants and Their Biological Activities
Compound Name | Structural Modification | Biological Activity |
---|---|---|
1-(2,3-Dihydrobenzofuran-7-yl)piperazine | Substituted at position 7 | Different receptor affinity |
1-(2-Methylbenzofuran-5-yl)methylpiperazine | Variation in methyl substitution pattern | Altered pharmacodynamics |
1-(Benzofuran-2-carboxylic acid)piperazine | Incorporates a carboxylic acid group | Increased solubility and bioactivity |
Properties
Molecular Formula |
C14H20N2O |
---|---|
Molecular Weight |
232.32 g/mol |
IUPAC Name |
1-[(2-methyl-2,3-dihydro-1-benzofuran-5-yl)methyl]piperazine |
InChI |
InChI=1S/C14H20N2O/c1-11-8-13-9-12(2-3-14(13)17-11)10-16-6-4-15-5-7-16/h2-3,9,11,15H,4-8,10H2,1H3 |
InChI Key |
XVDLFBGQAGRYJG-UHFFFAOYSA-N |
Canonical SMILES |
CC1CC2=C(O1)C=CC(=C2)CN3CCNCC3 |
Origin of Product |
United States |
Disclaimer and Information on In-Vitro Research Products
Please be aware that all articles and product information presented on BenchChem are intended solely for informational purposes. The products available for purchase on BenchChem are specifically designed for in-vitro studies, which are conducted outside of living organisms. In-vitro studies, derived from the Latin term "in glass," involve experiments performed in controlled laboratory settings using cells or tissues. It is important to note that these products are not categorized as medicines or drugs, and they have not received approval from the FDA for the prevention, treatment, or cure of any medical condition, ailment, or disease. We must emphasize that any form of bodily introduction of these products into humans or animals is strictly prohibited by law. It is essential to adhere to these guidelines to ensure compliance with legal and ethical standards in research and experimentation.