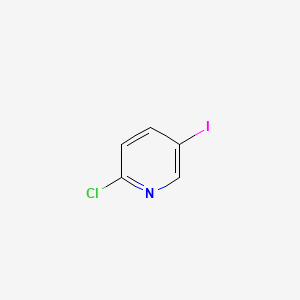
2-Chloro-5-iodopyridine
Overview
Description
2-Chloro-5-iodopyridine is a halo-substituted pyridine with the molecular formula C5H3ClIN. It is a crystalline powder or flakes that range in color from off-white to yellow-beige . This compound is used as a reagent in various chemical syntheses, including the multi-step synthesis of (±)-epibatidine .
Preparation Methods
2-Chloro-5-iodopyridine can be synthesized through several methods. One common synthetic route involves the iodination of 2-chloropyridine. This process typically includes the following steps:
Nitration: 2-Chloro-5-methylpyridine is subjected to nitration reactions.
Reduction: The nitrated product undergoes reduction reactions.
Diazotization: The reduced product is then diazotized.
Iodination: Finally, the diazotized product is iodinated to yield this compound.
Chemical Reactions Analysis
2-Chloro-5-iodopyridine undergoes various chemical reactions, including:
Substitution Reactions: It can participate in Suzuki coupling reactions with phenylboronic acid dimethyl ester to form 2-Chloro-5-phenylpyridine.
Heck Coupling Reactions: It can react with N-protected 2-azabicyclo[2.2.1]hept-5-enes to form exo-5- and exo-6-(6′-chloro-3′-pyridyl)-2-azabicyclo[2.2.1]heptanes.
Formation of Diaryliodonium Salts: It can be used in the synthesis of substituted diaryliodonium salts.
Scientific Research Applications
2-Chloro-5-iodopyridine is utilized in various scientific research applications:
Chemistry: It serves as a reagent in the synthesis of complex organic molecules, such as (±)-epibatidine.
Biology: It is used in the synthesis of biologically active compounds, including potential pharmaceuticals.
Medicine: The compound is involved in the development of new drugs and therapeutic agents.
Industry: It is used in the production of various chemical intermediates and specialty chemicals.
Mechanism of Action
The mechanism of action of 2-Chloro-5-iodopyridine involves its role as a versatile reagent in organic synthesis. It acts as both a nucleophile and an electrophile due to the presence of the chlorine and iodine atoms, respectively. This dual functionality allows it to participate in a wide range of chemical reactions, facilitating the formation of carbon-nitrogen and carbon-carbon bonds .
Comparison with Similar Compounds
2-Chloro-5-iodopyridine can be compared with other halo-substituted pyridines, such as:
2-Iodopyridine: This compound has a similar structure but lacks the chlorine atom.
3-Iodopyridine: This isomer has the iodine atom at the third position and is used in the synthesis of pyridine alkaloids.
2-Chloro-5-phenylpyridine: Formed via Suzuki coupling reactions, this compound is used in various organic syntheses.
This compound is unique due to its dual halogen substitution, which provides it with distinct reactivity and versatility in chemical synthesis.
Properties
IUPAC Name |
2-chloro-5-iodopyridine | |
---|---|---|
Source | PubChem | |
URL | https://pubchem.ncbi.nlm.nih.gov | |
Description | Data deposited in or computed by PubChem | |
InChI |
InChI=1S/C5H3ClIN/c6-5-2-1-4(7)3-8-5/h1-3H | |
Source | PubChem | |
URL | https://pubchem.ncbi.nlm.nih.gov | |
Description | Data deposited in or computed by PubChem | |
InChI Key |
QWLGCWXSNYKKDO-UHFFFAOYSA-N | |
Source | PubChem | |
URL | https://pubchem.ncbi.nlm.nih.gov | |
Description | Data deposited in or computed by PubChem | |
Canonical SMILES |
C1=CC(=NC=C1I)Cl | |
Source | PubChem | |
URL | https://pubchem.ncbi.nlm.nih.gov | |
Description | Data deposited in or computed by PubChem | |
Molecular Formula |
C5H3ClIN | |
Source | PubChem | |
URL | https://pubchem.ncbi.nlm.nih.gov | |
Description | Data deposited in or computed by PubChem | |
DSSTOX Substance ID |
DTXSID90402837 | |
Record name | 2-Chloro-5-iodopyridine | |
Source | EPA DSSTox | |
URL | https://comptox.epa.gov/dashboard/DTXSID90402837 | |
Description | DSSTox provides a high quality public chemistry resource for supporting improved predictive toxicology. | |
Molecular Weight |
239.44 g/mol | |
Source | PubChem | |
URL | https://pubchem.ncbi.nlm.nih.gov | |
Description | Data deposited in or computed by PubChem | |
CAS No. |
69045-79-0 | |
Record name | 2-Chloro-5-iodopyridine | |
Source | CAS Common Chemistry | |
URL | https://commonchemistry.cas.org/detail?cas_rn=69045-79-0 | |
Description | CAS Common Chemistry is an open community resource for accessing chemical information. Nearly 500,000 chemical substances from CAS REGISTRY cover areas of community interest, including common and frequently regulated chemicals, and those relevant to high school and undergraduate chemistry classes. This chemical information, curated by our expert scientists, is provided in alignment with our mission as a division of the American Chemical Society. | |
Explanation | The data from CAS Common Chemistry is provided under a CC-BY-NC 4.0 license, unless otherwise stated. | |
Record name | 2-Chloro-5-iodopyridine | |
Source | EPA DSSTox | |
URL | https://comptox.epa.gov/dashboard/DTXSID90402837 | |
Description | DSSTox provides a high quality public chemistry resource for supporting improved predictive toxicology. | |
Record name | 2-Chloro-5-iodopyridine | |
Source | European Chemicals Agency (ECHA) | |
URL | https://echa.europa.eu/information-on-chemicals | |
Description | The European Chemicals Agency (ECHA) is an agency of the European Union which is the driving force among regulatory authorities in implementing the EU's groundbreaking chemicals legislation for the benefit of human health and the environment as well as for innovation and competitiveness. | |
Explanation | Use of the information, documents and data from the ECHA website is subject to the terms and conditions of this Legal Notice, and subject to other binding limitations provided for under applicable law, the information, documents and data made available on the ECHA website may be reproduced, distributed and/or used, totally or in part, for non-commercial purposes provided that ECHA is acknowledged as the source: "Source: European Chemicals Agency, http://echa.europa.eu/". Such acknowledgement must be included in each copy of the material. ECHA permits and encourages organisations and individuals to create links to the ECHA website under the following cumulative conditions: Links can only be made to webpages that provide a link to the Legal Notice page. | |
Retrosynthesis Analysis
AI-Powered Synthesis Planning: Our tool employs the Template_relevance Pistachio, Template_relevance Bkms_metabolic, Template_relevance Pistachio_ringbreaker, Template_relevance Reaxys, Template_relevance Reaxys_biocatalysis model, leveraging a vast database of chemical reactions to predict feasible synthetic routes.
One-Step Synthesis Focus: Specifically designed for one-step synthesis, it provides concise and direct routes for your target compounds, streamlining the synthesis process.
Accurate Predictions: Utilizing the extensive PISTACHIO, BKMS_METABOLIC, PISTACHIO_RINGBREAKER, REAXYS, REAXYS_BIOCATALYSIS database, our tool offers high-accuracy predictions, reflecting the latest in chemical research and data.
Strategy Settings
Precursor scoring | Relevance Heuristic |
---|---|
Min. plausibility | 0.01 |
Model | Template_relevance |
Template Set | Pistachio/Bkms_metabolic/Pistachio_ringbreaker/Reaxys/Reaxys_biocatalysis |
Top-N result to add to graph | 6 |
Feasible Synthetic Routes
Q1: What makes 2-chloro-5-iodopyridine a useful building block in organic synthesis?
A: this compound is a versatile reagent due to the differing reactivity of the chlorine and iodine substituents. [, , ] This allows for regioselective reactions, meaning chemists can choose which substituent to target for a specific transformation. This is particularly useful in building more complex molecules in a controlled manner, as demonstrated in the synthesis of pyridin-3-yl C-nucleosides. []
Q2: What type of reactions have been explored with this compound using palladium catalysis?
A: Research has shown that this compound readily undergoes palladium-catalyzed cross-coupling reactions. [, ] Specifically, it participates in aminations, where the iodine atom is selectively replaced with an amine group, while the chlorine atom remains intact. [, ] This selectivity is key for further modifications and elaborations of the molecule. Additionally, it has been employed in Heck reactions, showcasing its versatility in constructing diverse chemical structures. []
Q3: What challenges are associated with palladium-catalyzed aminations of this compound and how are they addressed?
A: Achieving efficient palladium-catalyzed aminations on this compound requires careful consideration of reaction conditions. [, ] One challenge is the potential for competing reactions or slow reaction rates. Studies have found that using a large excess of cesium carbonate (Cs2CO3) in combination with a suitable palladium catalyst, such as Pd-BINAP, is crucial for accelerating the desired amination and achieving good selectivity. [, ]
Q4: Beyond C-nucleosides, what other applications might stem from the use of this compound in synthesis?
A: The regioselective functionalization of this compound opens doors to various applications. [, , ] For instance, it has been utilized in the synthesis of a tetracyclic oxanorbornane scaffold. [] This scaffold holds potential in medicinal chemistry due to its rigidity and high oxygen content, which could be beneficial for drug design and development. Further exploration of this compound's reactivity could lead to the development of new materials, catalysts, or biologically active compounds.
Disclaimer and Information on In-Vitro Research Products
Please be aware that all articles and product information presented on BenchChem are intended solely for informational purposes. The products available for purchase on BenchChem are specifically designed for in-vitro studies, which are conducted outside of living organisms. In-vitro studies, derived from the Latin term "in glass," involve experiments performed in controlled laboratory settings using cells or tissues. It is important to note that these products are not categorized as medicines or drugs, and they have not received approval from the FDA for the prevention, treatment, or cure of any medical condition, ailment, or disease. We must emphasize that any form of bodily introduction of these products into humans or animals is strictly prohibited by law. It is essential to adhere to these guidelines to ensure compliance with legal and ethical standards in research and experimentation.