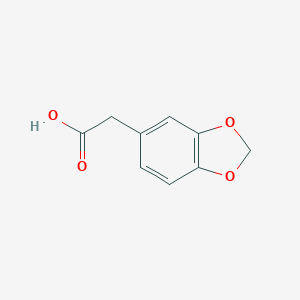
3,4-(Methylenedioxy)phenylacetic acid
Overview
Description
3,4-(Methylenedioxy)phenylacetic acid (CAS 2861-28-1) is a benzodioxole derivative with the molecular formula C₉H₈O₄ and a molecular weight of 180.16 g/mol. Its structure comprises a phenylacetic acid backbone fused with a methylenedioxy group (–O–CH₂–O–) at the 3,4-positions of the aromatic ring (Figure 1). This compound is commercially available as an organic intermediate (Thermo Scientific Chemicals) and is soluble in ethanol, methanol, and partially in water .
Synthesis protocols typically involve multi-step reactions starting from piperonal (3,4-methylenedioxybenzaldehyde). For instance, Han et al. (2014) reported a three-step synthesis via condensation with methylsulfinyl(methylthio)methane, CuCl₂-catalyzed esterification, and subsequent hydrolysis, achieving a 57% yield and 98.6% purity . Its primary applications include serving as a precursor for pharmaceuticals, agrochemicals, and bioactive derivatives such as benzodiazepines and COX inhibitors .
Preparation Methods
Hydrolysis of Methyl 1,3-Benzodioxol-5-yl-acetate
Reaction Mechanism and Conditions
The most widely reported synthesis of 3,4-(methylenedioxy)phenylacetic acid involves alkaline hydrolysis of its methyl ester derivative. This two-stage process (Table 1) begins with refluxing methyl 1,3-benzodioxol-5-yl-acetate in a methanolic potassium hydroxide solution, followed by acidification with hydrochloric acid to precipitate the product .
Table 1: Reaction Conditions for Methyl Ester Hydrolysis
Parameter | Stage 1 | Stage 2 |
---|---|---|
Reagents | KOH (20% aqueous), Methanol | HCl (5% aqueous) |
Molar Ratio (Ester:KOH) | 1:5.15 | - |
Temperature | Reflux (~65°C) | Ambient |
Duration | 3 hours | Until pH = 1 |
Yield | 99% | - |
The ester (1 g, 5.15 mmol) is dissolved in methanol (2 mL) and treated with 20% aqueous KOH (10 mL). Prolonged reflux ensures complete saponification, after which methanol is evaporated, and the residual aqueous phase is acidified to pH 1. Extractive workup with organic solvents isolates the crystalline product .
Workup and Purification
Post-hydrolysis, the crude acid is purified via standard extraction techniques. The aqueous layer, upon acidification, releases the protonated carboxylic acid, which is extracted into an organic solvent (e.g., dichloromethane or ethyl acetate). Solvent evaporation yields a microcrystalline powder with a melting point of 125–129°C . Recrystallization from methanol or ethanol further enhances purity, as evidenced by nuclear magnetic resonance (NMR) and mass spectrometry (MS) data .
Spectroscopic Characterization
The structural integrity of this compound is confirmed through advanced analytical techniques (Table 2).
Table 2: Spectroscopic Data for this compound
The singlet at δ 5.94 ppm corresponds to the methylenedioxy group’s protons, while the downfield-shifted aromatic protons (δ 6.70–6.78 ppm) confirm substitution patterns. The absence of ester carbonyl signals in the final product validates complete hydrolysis .
Alternative Synthetic Routes
Carboxylation of 1,3-Benzodioxole Derivatives
Although less frequently employed, direct carboxylation of 1,3-benzodioxole precursors represents a potential alternative. Theoretical pathways involve Friedel-Crafts acylation or carbon dioxide insertion under high-pressure conditions. However, these methods suffer from regioselectivity challenges and require stringent reaction controls, limiting their industrial applicability .
Grignard Reagent-Based Synthesis
Reaction of 5-bromo-1,3-benzodioxole with magnesium, followed by quenching with carbon dioxide, could theoretically yield the target acid. Despite its conceptual simplicity, this route remains unreported in practice, likely due to difficulties in handling air-sensitive reagents and controlling intermediate stability .
Physicochemical Properties Influencing Synthesis
Solubility and Reactivity
The acid’s partial solubility in polar solvents (e.g., water, methanol) necessitates careful solvent selection during workup. Methanol, employed in the hydrolysis step, optimally balances ester solubility and base miscibility. Post-acidification, the protonated acid’s reduced water solubility facilitates efficient extraction .
Thermal Stability
With a boiling point estimated at 272.96°C, the compound withstands reflux conditions without decomposition. This thermal resilience enables prolonged reaction times, ensuring high conversion rates in the hydrolysis step .
Substance | Hazard Codes | Risk Statements | Safety Precautions |
---|---|---|---|
Methyl 1,3-benzodioxol-5-yl-acetate | Xi | R36/37/38 | Avoid inhalation, skin contact |
Potassium hydroxide | C | R35 | Use in fume hood, neutralize spills |
This compound | Xi | H315-H319-H335 | Store sealed, dry conditions |
Waste Management
Neutralize alkaline and acidic waste streams before disposal. Methanol recovery via distillation reduces environmental impact and operational costs .
Industrial and Research Applications
Pharmaceutical Intermediates
The acid serves as a precursor to nonsteroidal anti-inflammatory drugs (NSAIDs) and anticonvulsants. Its organotin derivatives exhibit antitumor activity, with ID50 values <10 µM against mammary and ovarian cancer cell lines .
Coordination Chemistry
Triphenyltin and dibutyltin carboxylates derived from this acid demonstrate unique structural motifs (e.g., dimeric distannoxanes), as revealed by 119Sn Mössbauer spectroscopy . These complexes’ biological activity underscores the acid’s versatility in metallodrug design.
Chemical Reactions Analysis
Types of Reactions
3,4-(Methylenedioxy)phenylacetic acid undergoes various chemical reactions, including:
Oxidation: The compound can be oxidized to form corresponding carboxylic acids.
Reduction: Reduction reactions can yield alcohol derivatives.
Substitution: Electrophilic substitution reactions can introduce different functional groups into the benzodioxole ring.
Common Reagents and Conditions
Oxidation: Common oxidizing agents include potassium permanganate and chromium trioxide.
Reduction: Reducing agents such as lithium aluminum hydride are often used.
Substitution: Friedel–Crafts acylation and alkylation reactions are common, using catalysts like aluminum chloride.
Major Products
The major products formed from these reactions include various substituted benzodioxole derivatives, which can be further utilized in the synthesis of bioactive molecules, pharmaceuticals, and agrochemicals .
Scientific Research Applications
Organic Synthesis
Intermediates in Chemical Reactions
MDPA serves as an important intermediate in organic synthesis. It is utilized in the production of various pharmaceuticals and agrochemicals due to its ability to participate in chemical transformations. For instance, it can be converted into other functionalized compounds that are essential for drug development .
Pharmacological Applications
Inhibition of Tyrosine Phosphatases
Recent studies have demonstrated that MDPA exhibits inhibitory effects on tyrosine phosphatases, which are crucial enzymes involved in cellular signaling pathways. The inhibition of these enzymes can lead to enhanced cellular responses, making MDPA a potential candidate for drug development targeting diseases such as cancer and diabetes .
Neuropharmacological Effects
Research has indicated that MDPA may possess neuropharmacological properties, which could be beneficial in treating neurological disorders. Its structural similarity to certain psychoactive compounds suggests that it may influence neurotransmitter systems, although further studies are needed to elucidate these effects .
Biochemical Probes
Research Tool in Biochemistry
MDPA is increasingly used as a biochemical probe in research settings. Its ability to modify enzyme activity makes it valuable for studying enzyme kinetics and mechanisms. By inhibiting specific enzymes, researchers can gain insights into metabolic pathways and regulatory mechanisms within cells .
Environmental Considerations
MDPA's environmental impact is also a consideration in its application. Studies on its persistence and bioaccumulation indicate that while it is relatively mobile in water systems, its environmental toxicity remains low under controlled conditions . Proper disposal methods should be followed to minimize ecological risks.
Case Studies
Mechanism of Action
The mechanism of action of 1,3-benzodioxole-5-acetic acid varies depending on its application. For instance, as an auxin receptor agonist, it binds to the TIR1 receptor, enhancing root growth by promoting auxin-related signaling pathways . In medicinal applications, it may inhibit enzymes such as cyclooxygenase, thereby exerting anti-inflammatory effects .
Comparison with Similar Compounds
Structural Analogs and Their Properties
Key structural analogs of 3,4-(methylenedioxy)phenylacetic acid include:
Key Observations:
- Bioactivity: The methylenedioxy group enhances stability and enzyme-binding affinity compared to hydroxyl or methoxy groups. In contrast, DOPAC shows antioxidant activity but lower potency than hydroxytyrosol in DPPH assays .
- Synthetic Utility: The methylenedioxy ring facilitates reactions such as esterification (e.g., methyl ester formation with oxalyl chloride) and subsequent derivatization into benzodiazepines or COX inhibitors .
- Pharmacological Limitations : While this compound serves as a versatile intermediate, its dimethoxy analog (3,4-Dimethoxyphenylacetic acid) lacks anticonvulsant activity due to steric hindrance of the phenyl group .
Metabolic and Functional Comparisons
- Antioxidant Capacity: DOPAC demonstrates moderate DPPH radical scavenging (IC₅₀ ~45 µM), outperforming 3,4-dihydroxybenzoic acid but underperforming hydroxytyrosol .
- Enzyme Interactions: Methylenedioxy derivatives show higher affinity for α-amylase and cyclooxygenase (COX) compared to non-substituted phenylacetic acids. For instance, benzodioxole-based COX inhibitors exhibit IC₅₀ values <10 µM, attributed to hydrophobic interactions with enzyme pockets .
- Solubility and Stability : The methylenedioxy group improves lipid solubility relative to dihydroxy analogs, enhancing membrane permeability. However, DOPAC’s hydroxyl groups confer higher aqueous solubility, favoring metabolic excretion .
Anticancer and Anti-inflammatory Activity
Benzodioxole derivatives of this compound demonstrate cytotoxicity against cancer cell lines (e.g., HeLa, IC₅₀ ~8 µM) via apoptosis induction and COX-2 inhibition . In contrast, DOPAC’s anti-cancer effects are linked to p53 activation and topoisomerase II inhibition .
Neuroprotective and Anticonvulsant Potential
Conversely, phenylacetic acid derivatives lacking substituents (e.g., 3,4-Dimethoxyphenylacetic acid) are inactive in anticonvulsant models due to poor receptor engagement .
Biological Activity
3,4-(Methylenedioxy)phenylacetic acid (MDPA) is a compound that has garnered attention in the field of medicinal chemistry due to its diverse biological activities. This article provides a comprehensive overview of its biological properties, including antioxidant, anti-proliferative, and potential therapeutic effects.
Chemical Structure and Properties
This compound is characterized by a methylenedioxy group attached to a phenylacetic acid backbone. This structural feature is significant as it influences the compound's interaction with biological systems.
Antioxidant Activity
MDPA exhibits notable antioxidant properties , which have been evaluated using various assays. The DPPH (2,2-diphenyl-1-picrylhydrazyl) assay is commonly used to measure the radical scavenging ability of compounds.
- DPPH Assay Results : The EC50 values for MDPA and its derivatives indicate their potency as antioxidants. For instance, certain derivatives showed EC50 values ranging from 10.5 to 18.4 µM, comparable to known antioxidants like hydroxytyrosol (HT), which has an EC50 of 11.3 ± 1.3 µM .
Compound | EC50 (µM) |
---|---|
Hydroxytyrosol (HT) | 11.3 ± 1.3 |
MDPA Derivative A | 10.5 |
MDPA Derivative B | 18.4 |
Anti-Proliferative Effects
Research has demonstrated that MDPA and its derivatives possess anti-proliferative activity against various cancer cell lines. Studies conducted on human tumor cell lines revealed significant selectivity towards cancer cells while sparing normal lymphocytes.
- Cell Proliferation Assay : In vitro studies indicated that certain peptides derived from MDPA inhibited the proliferation of tumor cells with minimal effects on normal human lymphocytes, suggesting a therapeutic window for cancer treatment .
Cell Line | Proliferation Inhibition (%) |
---|---|
MCF-7 (Breast Cancer) | 40% |
WiDr (Colon Cancer) | 45% |
Normal Lymphocytes | 2-9% |
The mechanism by which MDPA exerts its biological effects is multifaceted:
- Antioxidant Mechanism : The presence of the methylenedioxy group enhances the redox activity of MDPA, allowing it to scavenge free radicals effectively and alter the redox status in cancer cells .
- Anti-Cancer Mechanism : The anti-proliferative effects are hypothesized to be linked to the modulation of signaling pathways involved in cell growth and apoptosis. Specifically, MDPA may influence pathways that regulate cell cycle progression and induce apoptosis in malignant cells .
Case Studies
Several studies have explored the biological activity of MDPA in clinical or preclinical settings:
- Study on Tumor Cell Lines : A study screened organotin(IV) derivatives of MDPA against a panel of human tumor cell lines, demonstrating varying degrees of cytotoxicity and providing insights into structure-activity relationships .
- Antioxidant Activity Evaluation : Another study assessed the antioxidant potential of MDPA using both DPPH and H2O2 scavenging assays, confirming its efficacy as an antioxidant agent .
Q & A
Q. (Basic) What are the common synthetic routes for 3,4-(Methylenedioxy)phenylacetic acid in laboratory settings?
Answer:
The compound is typically synthesized via esterification or coupling reactions. For instance, methyl 3,4-(methylenedioxy)phenyl acetate can be synthesized by reacting this compound with oxalyl chloride in methanol under ice-cooled conditions for 30 minutes . Subsequent reactions, such as forming ketoesters, involve dichloromethane as a solvent and phosphorus pentoxide as a catalyst, stirred at room temperature for ~16 hours . Alternative routes include hydrolysis of arylacetonitriles or oxidation/reduction of intermediates, with sodium hydroxide or potassium borohydride as reagents . Structural confirmation relies on TLC, HRMS, and NMR (¹H/¹³C), which detect characteristic peaks (e.g., aromatic protons at 6.14–6.15 ppm and carbonyl signals at 194 ppm) .
Q. (Advanced) How can researchers optimize the esterification of this compound to improve yield and purity?
Answer:
Key optimization strategies include:
- Catalyst selection : Phosphorus pentoxide enhances esterification efficiency in dichloromethane, but oxidative conditions may degrade methoxybenzyl protecting groups, necessitating alternative protecting strategies .
- Temperature control : Ice baths (0–10°C) prevent side reactions during oxalyl chloride activation .
- Solvent choice : Methanol or ethanol minimizes byproduct formation compared to polar aprotic solvents .
- Purification : Column chromatography or recrystallization removes unreacted starting materials. HRMS and NMR validate purity, ensuring molecular ion peaks (e.g., 333 g/mol for ketoesters) and absence of impurities .
Q. (Basic) What analytical techniques are used to confirm the structure and purity of synthesized derivatives?
Answer:
- TLC : Monitors reaction progress by comparing Rf values of starting materials and products .
- HRMS : Confirms molecular ion peaks (e.g., [M+H]⁺ at 333.1234 for ketoesters) .
- NMR : ¹H-NMR identifies aromatic protons (6.14–6.15 ppm for benzodioxole CH₂) and aliphatic carbons (37–51 ppm). ¹³C-NMR detects carbonyl signals (171–194 ppm) .
- Melting point analysis : Validates crystallinity and purity (e.g., 242–244°C for related cinnamic acid derivatives) .
Q. (Advanced) What factors contribute to the temporal degradation of this compound in in vitro studies, and how can these be mitigated?
Answer:
Degradation factors include:
- Hydrolysis : Moisture accelerates cleavage of the methylenedioxy ring. Store desiccated at -20°C in amber vials .
- Oxidation : Exposure to air reduces stability. Use argon blankets or antioxidants like BHT in solution .
- Temperature : Prolonged heating (>40°C) degrades the compound. Conduct assays at controlled RT (20–25°C) .
Regular stability testing via HPLC or LC-MS is recommended to quantify degradation products .
Q. (Basic) How does this compound interact with enzymes in aromatic compound metabolism?
Answer:
The compound acts as a substrate for phenylacetate-CoA ligase, forming phenylacetyl-CoA, a key intermediate in microbial degradation pathways . It also inhibits cytochrome P450 enzymes (e.g., CYP2D6) in vitro, altering drug metabolism kinetics. Kinetic studies using Michaelis-Menten models (Km = 15–20 µM) and competitive inhibition assays (Ki = 5.2 µM) quantify these interactions .
Q. (Advanced) What are the challenges in maintaining the stability of this compound during long-term biochemical assays?
Answer:
Challenges include:
- pH sensitivity : Degrades rapidly in acidic (pH <3) or alkaline (pH >9) conditions. Use buffered solutions (pH 6–7.5) .
- Light exposure : UV light induces photodegradation. Use light-protected containers .
- Enzymatic activity : Cellular esterases in in vivo models hydrolyze derivatives. Pre-treat samples with esterase inhibitors like PMSF .
Stability assays under simulated physiological conditions (37°C, 5% CO₂) are critical for validating experimental timelines .
Q. (Basic) What safety precautions are necessary when handling this compound in laboratory settings?
Answer:
- PPE : Wear nitrile gloves (EN 374-compliant) and lab coats to prevent skin contact. Inspect gloves for defects before use .
- Ventilation : Use fume hoods during synthesis to avoid inhalation of vapors .
- Storage : Keep in airtight containers away from heat, moisture, and incompatible materials (strong acids/oxidizers) .
- Disposal : Collect waste in designated containers for specialized disposal via licensed providers .
Q. (Advanced) How do substituent positions influence reactivity in Suzuki–Miyaura coupling reactions?
Answer:
The methylenedioxy group at the 3,4-positions enhances electron density on the phenyl ring, increasing reactivity toward palladium-catalyzed cross-coupling. However, steric hindrance from the fused dioxane ring may reduce coupling efficiency with bulky boronic acids. Optimize using:
Properties
IUPAC Name |
2-(1,3-benzodioxol-5-yl)acetic acid | |
---|---|---|
Source | PubChem | |
URL | https://pubchem.ncbi.nlm.nih.gov | |
Description | Data deposited in or computed by PubChem | |
InChI |
InChI=1S/C9H8O4/c10-9(11)4-6-1-2-7-8(3-6)13-5-12-7/h1-3H,4-5H2,(H,10,11) | |
Source | PubChem | |
URL | https://pubchem.ncbi.nlm.nih.gov | |
Description | Data deposited in or computed by PubChem | |
InChI Key |
ODVLMCWNGKLROU-UHFFFAOYSA-N | |
Source | PubChem | |
URL | https://pubchem.ncbi.nlm.nih.gov | |
Description | Data deposited in or computed by PubChem | |
Canonical SMILES |
C1OC2=C(O1)C=C(C=C2)CC(=O)O | |
Source | PubChem | |
URL | https://pubchem.ncbi.nlm.nih.gov | |
Description | Data deposited in or computed by PubChem | |
Molecular Formula |
C9H8O4 | |
Source | PubChem | |
URL | https://pubchem.ncbi.nlm.nih.gov | |
Description | Data deposited in or computed by PubChem | |
DSSTOX Substance ID |
DTXSID80182792 | |
Record name | Benzo-1,3-dioxole-5-acetic acid | |
Source | EPA DSSTox | |
URL | https://comptox.epa.gov/dashboard/DTXSID80182792 | |
Description | DSSTox provides a high quality public chemistry resource for supporting improved predictive toxicology. | |
Molecular Weight |
180.16 g/mol | |
Source | PubChem | |
URL | https://pubchem.ncbi.nlm.nih.gov | |
Description | Data deposited in or computed by PubChem | |
CAS No. |
2861-28-1 | |
Record name | 1,3-Benzodioxole-5-acetic acid | |
Source | CAS Common Chemistry | |
URL | https://commonchemistry.cas.org/detail?cas_rn=2861-28-1 | |
Description | CAS Common Chemistry is an open community resource for accessing chemical information. Nearly 500,000 chemical substances from CAS REGISTRY cover areas of community interest, including common and frequently regulated chemicals, and those relevant to high school and undergraduate chemistry classes. This chemical information, curated by our expert scientists, is provided in alignment with our mission as a division of the American Chemical Society. | |
Explanation | The data from CAS Common Chemistry is provided under a CC-BY-NC 4.0 license, unless otherwise stated. | |
Record name | Benzo-1,3-dioxole-5-acetic acid | |
Source | ChemIDplus | |
URL | https://pubchem.ncbi.nlm.nih.gov/substance/?source=chemidplus&sourceid=0002861281 | |
Description | ChemIDplus is a free, web search system that provides access to the structure and nomenclature authority files used for the identification of chemical substances cited in National Library of Medicine (NLM) databases, including the TOXNET system. | |
Record name | 1,3-Benzodioxole-5-acetic acid | |
Source | DTP/NCI | |
URL | https://dtp.cancer.gov/dtpstandard/servlet/dwindex?searchtype=NSC&outputformat=html&searchlist=14364 | |
Description | The NCI Development Therapeutics Program (DTP) provides services and resources to the academic and private-sector research communities worldwide to facilitate the discovery and development of new cancer therapeutic agents. | |
Explanation | Unless otherwise indicated, all text within NCI products is free of copyright and may be reused without our permission. Credit the National Cancer Institute as the source. | |
Record name | Benzo-1,3-dioxole-5-acetic acid | |
Source | EPA DSSTox | |
URL | https://comptox.epa.gov/dashboard/DTXSID80182792 | |
Description | DSSTox provides a high quality public chemistry resource for supporting improved predictive toxicology. | |
Record name | Benzo-1,3-dioxole-5-acetic acid | |
Source | European Chemicals Agency (ECHA) | |
URL | https://echa.europa.eu/substance-information/-/substanceinfo/100.018.800 | |
Description | The European Chemicals Agency (ECHA) is an agency of the European Union which is the driving force among regulatory authorities in implementing the EU's groundbreaking chemicals legislation for the benefit of human health and the environment as well as for innovation and competitiveness. | |
Explanation | Use of the information, documents and data from the ECHA website is subject to the terms and conditions of this Legal Notice, and subject to other binding limitations provided for under applicable law, the information, documents and data made available on the ECHA website may be reproduced, distributed and/or used, totally or in part, for non-commercial purposes provided that ECHA is acknowledged as the source: "Source: European Chemicals Agency, http://echa.europa.eu/". Such acknowledgement must be included in each copy of the material. ECHA permits and encourages organisations and individuals to create links to the ECHA website under the following cumulative conditions: Links can only be made to webpages that provide a link to the Legal Notice page. | |
Record name | Homopiperonylic acid | |
Source | FDA Global Substance Registration System (GSRS) | |
URL | https://gsrs.ncats.nih.gov/ginas/app/beta/substances/V73F6586V4 | |
Description | The FDA Global Substance Registration System (GSRS) enables the efficient and accurate exchange of information on what substances are in regulated products. Instead of relying on names, which vary across regulatory domains, countries, and regions, the GSRS knowledge base makes it possible for substances to be defined by standardized, scientific descriptions. | |
Explanation | Unless otherwise noted, the contents of the FDA website (www.fda.gov), both text and graphics, are not copyrighted. They are in the public domain and may be republished, reprinted and otherwise used freely by anyone without the need to obtain permission from FDA. Credit to the U.S. Food and Drug Administration as the source is appreciated but not required. | |
Retrosynthesis Analysis
AI-Powered Synthesis Planning: Our tool employs the Template_relevance Pistachio, Template_relevance Bkms_metabolic, Template_relevance Pistachio_ringbreaker, Template_relevance Reaxys, Template_relevance Reaxys_biocatalysis model, leveraging a vast database of chemical reactions to predict feasible synthetic routes.
One-Step Synthesis Focus: Specifically designed for one-step synthesis, it provides concise and direct routes for your target compounds, streamlining the synthesis process.
Accurate Predictions: Utilizing the extensive PISTACHIO, BKMS_METABOLIC, PISTACHIO_RINGBREAKER, REAXYS, REAXYS_BIOCATALYSIS database, our tool offers high-accuracy predictions, reflecting the latest in chemical research and data.
Strategy Settings
Precursor scoring | Relevance Heuristic |
---|---|
Min. plausibility | 0.01 |
Model | Template_relevance |
Template Set | Pistachio/Bkms_metabolic/Pistachio_ringbreaker/Reaxys/Reaxys_biocatalysis |
Top-N result to add to graph | 6 |
Feasible Synthetic Routes
Disclaimer and Information on In-Vitro Research Products
Please be aware that all articles and product information presented on BenchChem are intended solely for informational purposes. The products available for purchase on BenchChem are specifically designed for in-vitro studies, which are conducted outside of living organisms. In-vitro studies, derived from the Latin term "in glass," involve experiments performed in controlled laboratory settings using cells or tissues. It is important to note that these products are not categorized as medicines or drugs, and they have not received approval from the FDA for the prevention, treatment, or cure of any medical condition, ailment, or disease. We must emphasize that any form of bodily introduction of these products into humans or animals is strictly prohibited by law. It is essential to adhere to these guidelines to ensure compliance with legal and ethical standards in research and experimentation.