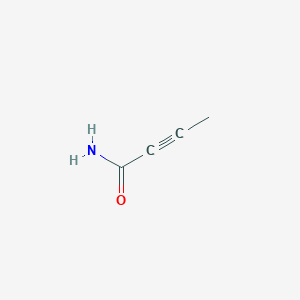
But-2-ynamide
Overview
Description
But-2-ynamide is an organic compound characterized by a four-carbon chain (but-) with a terminal triple bond (yne) at the second position and an amide functional group. Its general structure is RC≡C-C(=O)-NR'R'', where substituents on the nitrogen and carbon backbone influence its reactivity and applications. This compound derivatives are pivotal in synthetic chemistry, particularly in palladium-catalyzed asymmetric synthesis for constructing lactams and other bioactive heterocycles .
Key synthetic routes involve N-allylic substitution and cyclization reactions. For instance, (Z)-N-allylic but-2-ynamides are synthesized via palladium(II) catalysis, yielding stereochemically defined products critical for pharmaceutical intermediates . Structural characterization employs advanced techniques such as NMR (¹H, ¹³C), MS, IR, and HRMS to confirm regiochemistry and stereochemistry .
Preparation Methods
Synthetic Routes and Reaction Conditions
The synthesis of But-2-ynamide typically involves the coupling of an alkyne with an amide. One common method is the reaction of a haloenamide with an alkyne in the presence of a base. For example, the reaction of N-alkynylamide with a haloenamide under basic conditions can yield this compound . Another method involves the use of alkynylboron reagents and amides, which can be catalyzed by copper salts .
Industrial Production Methods
Industrial production of this compound can be achieved through scalable one-step synthetic methods from inexpensive starting materials. This approach not only reduces costs but also simplifies the purification process, making it suitable for large-scale production .
Chemical Reactions Analysis
Types of Reactions
But-2-ynamide undergoes a variety of chemical reactions, including:
Oxidation: This compound can be oxidized to form keteniminium ions, which are useful intermediates in organic synthesis.
Reduction: Reduction of this compound can yield amines or other reduced products depending on the conditions used.
Common Reagents and Conditions
Oxidation: Common oxidizing agents include peroxides and transition metal oxides.
Reduction: Reducing agents such as lithium aluminum hydride or hydrogen gas in the presence of a catalyst can be used.
Substitution: Nucleophiles such as amines, alcohols, and thiols can react with this compound under mild conditions.
Major Products
The major products formed from these reactions include keteniminium ions, amines, and various substituted ynamides .
Scientific Research Applications
But-2-ynamide is a compound with the alkyne group attached to a nitrogen atom, which has found applications across various scientific research fields . Its unique structure and reactivity have been harnessed in organic synthesis, peptide chemistry, and radical reactions .
Scientific Research Applications
Organic Synthesis
- Structural Reshuffling: But-2-ynamides can undergo photoinduced radical-triggered bond fission and structural reshuffling. This process allows for the creation of complex molecular architectures and the synthesis of otherwise inaccessible compounds .
- Chalcogen-Substituted Indole Derivatives: But-2-ynamides can be used as precursors to synthesize chalcogen-substituted indole derivatives, which are of interest in pharmaceutical chemistry .
- Radical Reactions: Ynamides can be used in radical reactions to create diverse compounds. For example, photocatalyzed generation of a radical on an ynamide substrate can be achieved through the single-electron reduction of a ketone .
Peptide Chemistry
- Peptide Bond Formation: Ynamides facilitate peptide bond formation, which has been expanded to fragment condensation and head-to-tail cyclization .
- Thiopeptide Synthesis: Ynamides can be employed as racemization-free coupling reagents for incorporating thioamide substitutions into the peptide backbone .
Elimination Reactions
- Ynamide Formation: Ynamides can be formed through elimination reactions from suitable precursors. For example, treating dichloroenamides with n-BuLi, followed by transmetallation with ZnBr2 and Negishi coupling with aryl iodides, yields ynamides .
- Building Blocks: Ynamides have been employed as building blocks in elimination protocols .
Other Applications
- Biological Studies: The unique reactivity of but-2-ynamides makes them useful in studying biological processes and developing new biochemical assays.
- Molecular Scaffolds: Ynamides can be used as precursors for complex molecular scaffolds in organic chemistry .
Case Studies
- Synthesis of Substituted Indole Derivatives: A model substrate N-(phenylethynyl)-N-(2-(phenylethynyl)phenyl)benzenesulfonamide with 4-methylbenzenesulfonyl iodide, when exposed to blue LED light, underwent radical-triggered ynamide bond fission and structural reshuffling, resulting in the formation of a single isomer, (E)-3-(1-iodo-2-phenyl-2-tosylvinyl)-2-phenyl-1-tosylindole .
- Photocatalyzed Radical Generation: In 2020, a group devised a method for photocatalyzed generation of a radical on an ynamide substrate through single-electron reduction of a ketone. Depending on whether the ynamides are derived from anilines or benzylamines, indoles or isoquinolines can be obtained, respectively, with generally good yields and functional group tolerance .
Mechanism of Action
The mechanism of action of But-2-ynamide involves its unique reactivity due to the polarized triple bond. This polarization allows this compound to participate in both nucleophilic and electrophilic reactions. The electron-withdrawing group on the nitrogen atom stabilizes the intermediate species formed during these reactions, facilitating various transformations . In biological systems, this compound derivatives can act as covalent inhibitors by forming stable bonds with target proteins, thereby modulating their activity .
Comparison with Similar Compounds
But-2-ynamide shares structural and functional similarities with other alkynamides and amide-containing compounds. Below is a comparative analysis based on substituent diversity, molecular properties, and applications.
Structural and Functional Comparison
Table 1: Structural Features of this compound and Analogues
Key Observations:
- Substituent Effects: Electron-withdrawing groups (e.g., pyridinyl in ) enhance electrophilicity at the triple bond, facilitating cycloaddition reactions. Bulky substituents (e.g., tosyl in ) influence stereoselectivity in asymmetric synthesis.
- Spectral Signatures: ¹H NMR chemical shifts for the alkyne proton (δ 2.5–3.0 ppm) and amide protons (δ 6.5–8.5 ppm) vary with substituents, aiding structural elucidation .
Physical and Chemical Properties
Melting/Boiling Points: Limited data exist for this compound itself, but derivatives with aromatic substituents (e.g., ) typically show higher melting points (>150°C) due to crystalline packing. Solubility: Polar aprotic solvents (e.g., DMF, DMSO) are preferred for most derivatives, while halogenated versions (e.g., ) show improved lipid solubility for membrane penetration .
Biological Activity
But-2-ynamide, a compound with the chemical formula C₄H₅NO, has garnered attention for its diverse biological activities, particularly in drug discovery and organic synthesis. This article explores its biological properties, synthesis methods, and potential applications based on current research findings.
Chemical Structure and Properties
This compound features a unique structure combining an alkyne and an amide group, which contributes to its reactivity and biological activity. The presence of the ynamide functionality allows it to mimic specific functional groups found in natural products, facilitating the synthesis of complex molecules.
1. Enzyme Inhibition
This compound has been identified as a significant electrophile in the development of covalent inhibitors targeting specific enzymes, notably Bruton’s tyrosine kinase (BTK). The compound's ability to form stable covalent bonds with nucleophilic residues in proteins enables it to modulate enzyme function effectively. This characteristic is crucial for designing targeted therapies in various diseases, including cancers where BTK plays a critical role.
2. Antimicrobial and Anticancer Properties
Research indicates that this compound and related compounds exhibit potential antimicrobial and anticancer activities. However, comprehensive studies are still needed to elucidate these effects fully. Preliminary findings suggest that these compounds may disrupt cellular processes in pathogens and cancer cells, highlighting their potential as therapeutic agents.
3. Protein Modification
This compound has shown promise in modifying cysteine residues in proteins. For instance, studies demonstrated that biotinylated derivatives of this compound could selectively modify cysteine-containing proteins without affecting other disulfide bonds. This selectivity is vital for developing antibody-drug conjugates (ADCs) and other targeted therapies .
Synthesis Methods
Several synthetic routes have been developed for preparing this compound, which include:
- Amination Reactions : Utilizing copper or palladium catalysts to facilitate the transformation of various nitrogen nucleophiles into ynamides.
- Cycloaddition Reactions : Employing this compound as a precursor for synthesizing complex cyclic structures through cycloaddition reactions .
Case Study 1: Inhibition of SARS-CoV-2 Protease
A study evaluated the efficacy of this compound derivatives as inhibitors of the SARS-CoV-2 3CL protease. The most reactive compounds demonstrated IC50 values significantly lower than non-covalent inhibitors, indicating that modifications to the ynamide structure could enhance antiviral activity .
Case Study 2: Enzyme Interaction Studies
In another investigation, this compound was used to probe enzyme interactions, revealing its potential as a covalent modifier that influences enzyme activity through irreversible binding mechanisms. This study emphasized its relevance in pharmacological research and drug design .
Comparison with Related Compounds
The following table summarizes key features of this compound compared to related compounds:
Compound Name | Structure Features | Unique Aspects |
---|---|---|
Butyne | Simple alkyne | Lacks amide functionality; primarily a building block. |
Ynamides (General) | Alkyne bonded to nitrogen | Diverse reactivity; this compound has specific electrophilic properties. |
N-Benzyl-N-(cyanomethyl)this compound | Contains cyano group | Enhanced reactivity due to additional functional groups; used in complex synthesis. |
N-(1-Methyl-2-phenylpiperidin-4-yl)this compound | Contains piperidine moiety | Increased complexity; potential for different biological activities. |
Q & A
Basic Research Questions
Q. What experimental methodologies are recommended for characterizing the receptor-binding affinity of But-2-ynamide in neurotransmitter systems?
To assess receptor affinity, employ radioligand binding assays using purified dopamine (D2/D3), serotonin (5-HT1A/2A), and norepinephrine (NET) receptors. Competitive inhibition experiments with labeled ligands (e.g., [³H]-spiperone for serotonin receptors) can quantify dissociation constants (Kd). Include saturation and displacement curves to validate specificity. For functional activity, pair binding assays with cAMP accumulation or calcium flux assays in transfected cell lines .
Q. What are the standard protocols for synthesizing and purifying this compound to ensure reproducibility in neuropharmacological studies?
Synthesis typically involves coupling the but-2-ynoic acid moiety to the piperidine-ethylamine backbone via carbodiimide-mediated amidation. Purify the product using column chromatography (silica gel, ethyl acetate/hexane gradient) and confirm purity (>98%) via HPLC with UV detection at 254 nm. Structural validation requires ¹H/¹³C NMR and high-resolution mass spectrometry (HRMS) to resolve potential stereoisomers .
Q. How can researchers mitigate batch-to-batch variability in this compound’s pharmacological effects?
Standardize synthesis protocols and validate each batch with:
- Purity assays : HPLC, NMR.
- Stability tests : Accelerated degradation studies under varying pH/temperature.
- Biological validation : Consistent EC50/IC50 values in receptor-binding assays across batches .
Advanced Research Questions
Q. What experimental designs resolve contradictions in reported neurotransmitter modulation by this compound (e.g., dopamine elevation vs. no effect)?
Contradictions may arise from model-specific factors (e.g., in vivo vs. in vitro systems). To address this:
- Cross-validate models : Compare microdialysis (in vivo dopamine release) with ex vivo brain slice electrophysiology.
- Control variables : Strain/species differences, dosing regimens, and pharmacokinetic profiles (e.g., blood-brain barrier penetration).
- Statistical rigor : Use ANOVA with post-hoc tests to assess inter-study variability .
Q. How can researchers optimize in vivo studies to isolate this compound’s effects on specific neurotransmitter pathways?
- Knockout models : Use dopamine transporter (DAT) or serotonin transporter (SERT) knockout mice to isolate receptor-level effects.
- Pharmacological blockade : Co-administer selective antagonists (e.g., haloperidol for D2 receptors) during behavioral assays.
- Temporal resolution : Employ fast-scan cyclic voltammetry (FSCV) for real-time neurotransmitter monitoring in target brain regions .
Q. What advanced analytical techniques are critical for elucidating this compound’s off-target interactions?
- Proteome-wide screening : Use affinity chromatography coupled with mass spectrometry (AfC-MS) to identify non-canonical binding partners.
- Molecular dynamics simulations : Model ligand-receptor interactions to predict off-target sites (e.g., adrenergic or histaminergic receptors).
- Functional genomics : CRISPR-Cas9 screens to identify genes modulating this compound’s efficacy .
Q. How should researchers design dose-response studies to account for this compound’s non-linear pharmacokinetics?
- Pilot pharmacokinetics : Measure plasma and brain concentrations via LC-MS/MS at multiple timepoints.
- Dose-ranging : Test logarithmic increments (e.g., 0.1–100 mg/kg) to capture threshold effects.
- Mechanistic modeling : Apply Hill equations to distinguish receptor saturation from compensatory pathways .
Q. Methodological Guidelines for Data Interpretation
- Contradiction analysis : Use principal contradiction frameworks to prioritize variables (e.g., receptor density vs. ligand affinity) driving divergent results .
- Data transparency : Archive raw datasets (e.g., binding curves, chromatograms) in repositories like PubChem or Zenodo for independent validation .
Properties
IUPAC Name |
but-2-ynamide | |
---|---|---|
Details | Computed by LexiChem 2.6.6 (PubChem release 2019.06.18) | |
Source | PubChem | |
URL | https://pubchem.ncbi.nlm.nih.gov | |
Description | Data deposited in or computed by PubChem | |
InChI |
InChI=1S/C4H5NO/c1-2-3-4(5)6/h1H3,(H2,5,6) | |
Details | Computed by InChI 1.0.5 (PubChem release 2019.06.18) | |
Source | PubChem | |
URL | https://pubchem.ncbi.nlm.nih.gov | |
Description | Data deposited in or computed by PubChem | |
InChI Key |
STSBIYSZXHSXHH-UHFFFAOYSA-N | |
Details | Computed by InChI 1.0.5 (PubChem release 2019.06.18) | |
Source | PubChem | |
URL | https://pubchem.ncbi.nlm.nih.gov | |
Description | Data deposited in or computed by PubChem | |
Canonical SMILES |
CC#CC(=O)N | |
Details | Computed by OEChem 2.1.5 (PubChem release 2019.06.18) | |
Source | PubChem | |
URL | https://pubchem.ncbi.nlm.nih.gov | |
Description | Data deposited in or computed by PubChem | |
Molecular Formula |
C4H5NO | |
Details | Computed by PubChem 2.1 (PubChem release 2019.06.18) | |
Source | PubChem | |
URL | https://pubchem.ncbi.nlm.nih.gov | |
Description | Data deposited in or computed by PubChem | |
Molecular Weight |
83.09 g/mol | |
Details | Computed by PubChem 2.1 (PubChem release 2021.05.07) | |
Source | PubChem | |
URL | https://pubchem.ncbi.nlm.nih.gov | |
Description | Data deposited in or computed by PubChem | |
Disclaimer and Information on In-Vitro Research Products
Please be aware that all articles and product information presented on BenchChem are intended solely for informational purposes. The products available for purchase on BenchChem are specifically designed for in-vitro studies, which are conducted outside of living organisms. In-vitro studies, derived from the Latin term "in glass," involve experiments performed in controlled laboratory settings using cells or tissues. It is important to note that these products are not categorized as medicines or drugs, and they have not received approval from the FDA for the prevention, treatment, or cure of any medical condition, ailment, or disease. We must emphasize that any form of bodily introduction of these products into humans or animals is strictly prohibited by law. It is essential to adhere to these guidelines to ensure compliance with legal and ethical standards in research and experimentation.