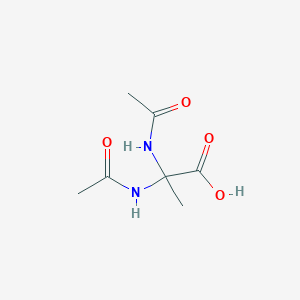
2,2-Diacetamidopropanoic acid
- Click on QUICK INQUIRY to receive a quote from our team of experts.
- With the quality product at a COMPETITIVE price, you can focus more on your research.
Overview
Description
2,2-Diacetamidopropanoic acid is a chemical compound with the molecular formula C7H12N2O4 . It is not classified as a hazardous compound .
Molecular Structure Analysis
The molecular structure of 2,2-Diacetamidopropanoic acid consists of 7 carbon atoms, 12 hydrogen atoms, 2 nitrogen atoms, and 4 oxygen atoms . The molecular weight is 188.18100 .
Scientific Research Applications
Bioconjugation and Labeling
Bioconjugation involves attaching molecules to biomolecules (e.g., proteins, peptides, or nucleic acids). 2,2-Diacetamidopropanoic acid can serve as a linker or spacer in bioconjugates. Researchers use it to attach fluorophores, enzymes, or other functional groups to biomolecules for imaging, diagnostics, or targeted drug delivery.
These applications highlight the versatility of 2,2-diacetamidopropanoic acid across diverse scientific disciplines. Researchers continue to explore its potential, and its unique properties make it an intriguing compound for future investigations . If you need further details or additional applications, feel free to ask!
Future Directions
properties
IUPAC Name |
2,2-diacetamidopropanoic acid |
Source
|
---|---|---|
Source | PubChem | |
URL | https://pubchem.ncbi.nlm.nih.gov | |
Description | Data deposited in or computed by PubChem | |
InChI |
InChI=1S/C7H12N2O4/c1-4(10)8-7(3,6(12)13)9-5(2)11/h1-3H3,(H,8,10)(H,9,11)(H,12,13) |
Source
|
Source | PubChem | |
URL | https://pubchem.ncbi.nlm.nih.gov | |
Description | Data deposited in or computed by PubChem | |
InChI Key |
XSWWTBWNVZPJBQ-UHFFFAOYSA-N |
Source
|
Source | PubChem | |
URL | https://pubchem.ncbi.nlm.nih.gov | |
Description | Data deposited in or computed by PubChem | |
Canonical SMILES |
CC(=O)NC(C)(C(=O)O)NC(=O)C |
Source
|
Source | PubChem | |
URL | https://pubchem.ncbi.nlm.nih.gov | |
Description | Data deposited in or computed by PubChem | |
Molecular Formula |
C7H12N2O4 |
Source
|
Source | PubChem | |
URL | https://pubchem.ncbi.nlm.nih.gov | |
Description | Data deposited in or computed by PubChem | |
DSSTOX Substance ID |
DTXSID10278793 |
Source
|
Record name | 2,2-diacetamidopropanoic acid | |
Source | EPA DSSTox | |
URL | https://comptox.epa.gov/dashboard/DTXSID10278793 | |
Description | DSSTox provides a high quality public chemistry resource for supporting improved predictive toxicology. | |
Molecular Weight |
188.18 g/mol |
Source
|
Source | PubChem | |
URL | https://pubchem.ncbi.nlm.nih.gov | |
Description | Data deposited in or computed by PubChem | |
Product Name |
2,2-Diacetamidopropanoic acid | |
CAS RN |
98337-17-8 |
Source
|
Record name | 2,2-diacetamidopropanoic acid | |
Source | EPA DSSTox | |
URL | https://comptox.epa.gov/dashboard/DTXSID10278793 | |
Description | DSSTox provides a high quality public chemistry resource for supporting improved predictive toxicology. | |
Disclaimer and Information on In-Vitro Research Products
Please be aware that all articles and product information presented on BenchChem are intended solely for informational purposes. The products available for purchase on BenchChem are specifically designed for in-vitro studies, which are conducted outside of living organisms. In-vitro studies, derived from the Latin term "in glass," involve experiments performed in controlled laboratory settings using cells or tissues. It is important to note that these products are not categorized as medicines or drugs, and they have not received approval from the FDA for the prevention, treatment, or cure of any medical condition, ailment, or disease. We must emphasize that any form of bodily introduction of these products into humans or animals is strictly prohibited by law. It is essential to adhere to these guidelines to ensure compliance with legal and ethical standards in research and experimentation.