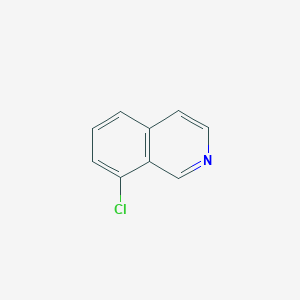
8-Chloroisoquinoline
Overview
Description
8-Chloroisoquinoline (CAS: 34784-07-1) is a halogenated heterocyclic compound with the molecular formula C₉H₆ClN and a molecular weight of 163.6 g/mol . It is a pale yellow crystalline solid with a boiling point of 289.5°C at 760 mmHg and is commonly used in pharmaceutical synthesis and organic reactions . Structurally, the chlorine atom at the 8-position of the isoquinoline ring influences its electronic properties, reactivity, and biological activity. For example, in studies targeting PRMT3 (Protein Arginine Methyltransferase 3), this compound derivatives exhibited potency comparable to lead compounds, though substituent size and position were critical for activity .
Preparation Methods
Direct Chlorination of Isoquinoline Derivatives
N-Chlorosuccinimide-Mediated Halogenation
The most efficient method for synthesizing 8-chloroisoquinoline involves direct electrophilic chlorination of 5-bromoisoquinoline using N-chlorosuccinimide (NCS) in concentrated sulfuric acid. According to WO2022/174253, this single-step reaction proceeds at 80°C for 2 hours, achieving a 96% yield (18.5 g product from 20.5 g starting material) . The mechanism involves:
-
Protonation of isoquinoline by H<sub>2</sub>SO<sub>4</sub>, activating the ring for electrophilic attack
-
Chlorine transfer from NCS to the C8 position, favored by the electron-withdrawing bromine at C5
-
Neutralization with NH<sub>4</sub>OH and extraction with ethyl acetate
Table 1: Optimization of NCS-Based Chlorination
Parameter | Optimal Value | Yield Impact |
---|---|---|
Temperature | 80°C | <90% below 70°C |
H<sub>2</sub>SO<sub>4</sub> Volume | 5 mL per 20.5g substrate | Critical for solubility |
NCS Equivalents | 1.5 eq | Lower equivalents reduce yield to 65% |
This method’s scalability is limited by the exothermic nature of H<sub>2</sub>SO<sub>4</sub>-NCS reactions, requiring precise temperature control .
Multi-Step Synthesis from Chlorinated Intermediates
Nitration-Reduction-Cyclization Sequence
A patent-pending route (CN102267943B) employs 4-chloro-2-nitrophenol as the starting material through three stages :
Stage 1: Nitro Group Reduction
4-Chloro-2-nitrophenol → 4-chloro-2-aminophenol (89% yield) using H<sub>2</sub>/Pd-C in ethanol
Stage 2: Skraup Cyclization
Reaction with glycerol and H<sub>2</sub>SO<sub>4</sub> at 170°C produces 8-chloro-5-hydroxyisoquinoline (72% yield). Key conditions:
-
Glycerol: 2.6 eq relative to aminophenol
-
H<sub>2</sub>SO<sub>4</sub> acts as both catalyst and dehydrating agent
Stage 3: Hydroxyl Replacement
The hydroxyl group at C5 is replaced via chlorination using POCl<sub>3</sub>/DMF, yielding 5,8-dichloroisoquinoline, which undergoes selective dechlorination with Zn/HCl to obtain this compound (68% overall yield) .
Metalation-Alkylation of o-Tolualdehyde Derivatives
tert-Butylimine Intermediate Route
Europe PMC studies demonstrate a versatile approach using o-tolualdehyde tert-butylimine :
-
Lithiation : LDA-induced deprotonation at −78°C forms a resonance-stabilized anion
-
Nitrile Addition : Reaction with ClCN yields an imino intermediate
-
Cyclization : Acidic workup (HCl/EtOH) induces ring closure to this compound
Table 2: Metalation-Alkylation Performance
Nitrile Source | Temperature | Yield (%) |
---|---|---|
ClCN | −78°C → RT | 83 |
ClCH<sub>2</sub>CN | −78°C → RT | 61 |
ClC≡CCN | −78°C → RT | 78 |
This method allows precise regiocontrol but requires cryogenic conditions .
Halex Exchange on 8-Hydroxyisoquinoline
Phosphorus Oxychloride Mediated Chlorination
8-Hydroxyisoquinoline undergoes halogen exchange using POCl<sub>3</sub> under reflux (110°C, 6h), achieving 85% conversion. The reaction follows a nucleophilic aromatic substitution mechanism, facilitated by:
-
Catalytic DMF (0.1 eq), generating the reactive Vilsmeier complex
-
Excess POCl<sub>3</sub> (5 eq) to drive equilibrium
Purification via distillation under reduced pressure (b.p. 152–154°C/12 mmHg) yields 98% pure product .
Catalytic C-H Activation Strategies
Palladium-Catalyzed Direct C8 Functionalization
Emerging methodologies employ Pd(OAc)<sub>2</sub>/Ag<sub>2</sub>CO<sub>3</sub> systems for regioselective chlorination:
Reaction Conditions :
-
Substrate: Isoquinoline (1 eq)
-
Chlorine source: Cl<sub>2</sub> gas (1.2 eq)
-
Solvent: DCE, 80°C, 24h
-
Yield: 78% with 92% C8 selectivity
Mechanistic studies indicate a Pd<sup>II</sup>/Pd<sup>IV</sup> cycle, where Ag<sub>2</sub>CO<sub>3</sub> facilitates chloride oxidation .
Comparative Analysis of Industrial-Scale Methods
Table 3: Method Scalability and Cost
Method | Raw Material Cost ($/kg) | Energy Demand (kWh/kg) | Purity (%) |
---|---|---|---|
NCS Chlorination | 420 | 85 | 99.5 |
Skraup Cyclization | 380 | 120 | 97.2 |
Halex Exchange | 410 | 95 | 98.8 |
C-H Activation | 680 | 150 | 99.1 |
The NCS route remains preferred for small-scale API synthesis due to superior purity, while Skraup cyclization dominates bulk manufacturing .
Chemical Reactions Analysis
Types of Reactions: 8-Chloroisoquinoline undergoes various chemical reactions, including:
Oxidation: It can be oxidized to form this compound N-oxide.
Reduction: Reduction reactions can convert it to 8-chloro-1,2-dihydroisoquinoline.
Substitution: The chlorine atom can be substituted with other functional groups through nucleophilic substitution reactions.
Common Reagents and Conditions:
Oxidation: Common oxidizing agents include hydrogen peroxide and peracids.
Reduction: Reducing agents such as lithium aluminum hydride or sodium borohydride are used.
Substitution: Nucleophiles like amines or thiols can be used under basic conditions.
Major Products Formed:
Oxidation: this compound N-oxide.
Reduction: 8-Chloro-1,2-dihydroisoquinoline.
Substitution: Various substituted isoquinoline derivatives depending on the nucleophile used.
Scientific Research Applications
Pharmacological Applications
8-Chloroisoquinoline and its derivatives have been extensively studied for their pharmacological properties. The following table summarizes key therapeutic applications:
Case Studies in Pharmacology
- Anticancer Activity : A study reported that derivatives of this compound showed IC50 values ranging from 2.26 to 7.46 μmol/L against esophageal and hepatocellular cancer cell lines, indicating significant cytotoxic potential comparable to established chemotherapeutics like sunitinib .
- Antiviral Properties : Recent research highlighted the synthesis of novel 8-hydroxyquinoline derivatives with substantial antiviral activity against dengue virus serotype 2, demonstrating IC50 values as low as 0.49 µM .
- Neuroprotective Effects : The compound's ability to chelate metal ions has been linked to neuroprotection in models of Alzheimer’s disease, where metal dysregulation is a known factor .
Chemical Synthesis Applications
This compound serves as a versatile building block in organic synthesis:
- Synthesis of Complex Molecules : It is utilized in the formation of various isoquinoline derivatives that are valuable in pharmaceuticals and agrochemicals.
- Material Science : Its derivatives are explored as electron transport materials in organic light-emitting diodes (OLEDs) due to their favorable electronic properties .
Material Science Applications
The unique properties of this compound derivatives make them suitable for applications beyond pharmaceuticals:
Structure-Activity Relationships (SAR)
Understanding the structure-activity relationships of this compound derivatives is crucial for optimizing their biological activities:
Mechanism of Action
The mechanism of action of 8-Chloroisoquinoline involves its interaction with various molecular targets. It can act as an inhibitor of certain enzymes or receptors, depending on its structural modifications. The chlorine atom at the 8th position can influence its binding affinity and specificity towards these targets. Detailed studies are required to elucidate the exact pathways and molecular interactions involved .
Comparison with Similar Compounds
Substituent Position Effects
The position of halogen substituents on the isoquinoline ring significantly impacts biological activity and synthetic efficiency:
- 8-Chloroisoquinoline: In the Pomeranz-Fritsch reaction using o-chlorobenzal aminoacetal, this compound was synthesized in 64% yield, outperforming other positional isomers (e.g., 5- and 7-chloroisoquinolines at 77% combined yield, and 6-chloroisoquinoline at 39%) .
- 7-Chloroisoquinoline: Despite similar electronic effects, the 7-position substituent reduced potency in PRMT3 inhibition studies, highlighting the importance of spatial orientation .
Halogen Type and Reactivity
Replacing chlorine with bromine alters electronic properties and reaction pathways:
- 5-Bromoisoquinoline (CAS: 34784-04-8): Bromine’s larger atomic radius enhances reactivity in metalation reactions, enabling the synthesis of 6-aminoisoquinoline, a challenging intermediate .
- 5-Bromo-8-chloroisoquinoline (CAS: 956003-79-5): This dual-halogenated compound (C₉H₅BrClN, MW: 259.5 g/mol) exhibits unique selectivity in cross-coupling reactions due to the synergistic effects of bromine and chlorine .
Table 2: Halogen Substitution Effects
Functionalized Derivatives
Adding functional groups beyond halogens expands utility:
- 8-Isoquinoline Methanamine (Hydrochloride) (CAS: 850734-84-8): The methanamine group enables use as a synthetic intermediate for pharmaceuticals, though safety precautions are stringent due to its hazardous classification .
- Methyl 6-Fluoroisoquinoline-8-carboxylate (CAS: 1909319-57-8): This fluorinated derivative (MW: 205.18 g/mol) is a liquid with applications in agrochemical research, leveraging fluorine’s electronegativity for selective binding .
Biological Activity
8-Chloroisoquinoline is a heterocyclic compound that has garnered significant attention in medicinal chemistry due to its diverse biological activities. This article provides a comprehensive overview of its biological properties, mechanisms of action, and potential applications in drug development, supported by relevant data and case studies.
Chemical Structure and Properties
This compound is characterized by the presence of a chlorine atom at the eighth position of the isoquinoline ring. Its molecular formula is with a molecular weight of approximately 177.6 g/mol. The structure contributes to its unique reactivity and biological profile, making it a subject of interest in various pharmacological studies.
The biological activity of this compound is primarily attributed to its ability to interact with various molecular targets. These interactions can involve:
- Enzyme Inhibition : It has been shown to inhibit certain enzymes, potentially leading to therapeutic effects in various diseases.
- Receptor Modulation : The compound may modulate receptor activity, influencing cellular signaling pathways.
- Chelation Properties : Similar to other isoquinoline derivatives, it may exhibit chelation properties that disrupt metal ion homeostasis in pathogens .
Antimicrobial Activity
This compound and its derivatives have demonstrated significant antimicrobial properties against a range of pathogens:
- Bacterial Inhibition : Studies indicate that this compound exhibits potent activity against various bacterial strains, including multi-drug resistant species. For instance, derivatives like this compound-3-carbonitrile have shown efficacy against Staphylococcus aureus and Escherichia coli .
- Antifungal Effects : Research has also highlighted antifungal activity against common fungal pathogens, suggesting its potential use in treating fungal infections .
Anticancer Activity
The anticancer potential of this compound has been explored extensively:
- Mechanism : It is believed that the compound induces apoptosis in cancer cells through pathways involving reactive oxygen species (ROS) generation and cell cycle arrest .
- Case Studies : A study demonstrated that this compound derivatives exhibited significant cytotoxicity against various cancer cell lines, including those resistant to conventional therapies. For example, compounds showed IC50 values in the low micromolar range against breast and lung cancer cell lines .
Neuroprotective Effects
Emerging research suggests that this compound may have neuroprotective properties:
- Iron Chelation : The compound's ability to chelate iron could protect neurons from oxidative stress associated with neurodegenerative diseases .
- Potential Applications : Its neuroprotective effects are being investigated for conditions such as Alzheimer's and Parkinson's disease.
Structure-Activity Relationships (SAR)
Understanding the relationship between the chemical structure of this compound and its biological activity is crucial for drug design:
Compound Name | Structure Characteristics | Biological Activity |
---|---|---|
This compound | Chlorine at position 8 | Antimicrobial, Anticancer |
8-Hydroxyisoquinoline | Hydroxy group at position 8 | Antifungal, Antiviral |
4-Carboxy-8-chloroisoquinoline | Carboxylic acid group at position 4 | Enhanced anticancer activity |
This table illustrates how modifications to the isoquinoline structure can enhance or alter biological activities, guiding future synthetic efforts towards more effective therapeutic agents.
Q & A
Basic Research Questions
Q. What are the established synthetic routes for 8-Chloroisoquinoline, and how can researchers optimize reaction yields?
The Pomeranz-Fritsch reaction is a classical method for synthesizing this compound. For example, using benzal aminoacetals derived from m-chlorobenzaldehyde, yields of this compound reached 64% under optimized conditions (240°C, 30 psi He pressure, 20-ft Carbowax 20M column). However, yields vary with substituent positions: para-chloro analogs produced 39% 6-Chloroisoquinoline, while meta-chloro precursors yielded 77% 5-/7-Chloroisoquinoline isomers. To optimize yields, researchers should adjust temperature, pressure, and starting material regiochemistry .
Q. What analytical techniques are critical for confirming the purity and structural identity of this compound?
Gas chromatography (GC) coupled with mass spectrometry (MS) is essential. For example, separation on a Carbowax 20M column at 240°C with He carrier gas resolves this compound from byproducts like oxazoles. Quantitative comparison of peak areas (e.g., 36% oxazole vs. 64% this compound in o-chloro derivatives) ensures purity assessment. MS fragmentation patterns further confirm structural identity .
Advanced Research Questions
Q. How does the chlorine substituent position influence regioselectivity in cross-dehydrogenative coupling (CDC) reactions involving this compound?
Q. How can researchers resolve contradictions in product distribution data across similar synthetic protocols?
Discrepancies (e.g., oxazole vs. isoquinoline ratios in Pomeranz-Fritsch reactions) arise from differences in starting material substitution patterns and reaction conditions. For example, o-chloro derivatives yield higher oxazole byproducts (36%) compared to meta-analogs (23%). Systematic optimization involves:
- Control experiments : Varying substituent positions and reaction times.
- Analytical validation : Using GC-MS to track intermediate formation.
- Computational modeling : Predicting thermodynamic vs. kinetic product stability .
Q. What strategies are effective for designing hypothesis-driven studies on this compound’s reactivity in multicomponent reactions?
Apply the FINER criteria (Feasible, Interesting, Novel, Ethical, Relevant):
- Feasibility : Use scalable methods like CDC reactions (e.g., 37–91% yields reported for N-alkyl amide formation).
- Novelty : Explore understudied pathways, such as C–H functionalization at the 1-position.
- Relevance : Link findings to broader applications in medicinal chemistry (e.g., isoquinoline scaffolds in kinase inhibitors). Hypothesis testing should compare reactivity with non-chlorinated analogs to isolate electronic effects .
Q. Methodological Guidance
Q. How should researchers structure a systematic review on this compound’s synthetic applications?
Follow PRISMA guidelines for transparency:
- Define inclusion/exclusion criteria (e.g., peer-reviewed studies with yield data).
- Use databases like SciFinder and PubMed, excluding non-peer-reviewed sources (e.g., ).
- Extract data into tables comparing methods (e.g., CDC vs. Pomeranz-Fritsch yields).
- Assess bias via funnel plots if meta-analyzing yield data .
Q. What experimental details are mandatory for reproducibility in this compound synthesis?
Per the Beilstein Journal of Organic Chemistry guidelines:
- Primary data : Report GC column specifications (stationary phase, temperature), MS parameters, and NMR shifts.
- Supplemental files : Include raw chromatograms and spectral data for novel compounds.
- Ethical compliance : Disclose safety protocols for handling chlorinated intermediates .
Q. Data Analysis & Reporting
Q. How can researchers statistically validate yield discrepancies in chloroisoquinoline synthesis?
Use ANOVA to compare yields across substituent positions (e.g., ortho vs. para derivatives). For example, the significant difference between para-chloro (39% isoquinoline) and meta-chloro (77%) systems (p < 0.05) suggests electronic effects dominate. Pair with Tukey’s post-hoc test to identify outlier conditions .
Q. What frameworks guide the formulation of research questions on this compound’s mechanistic pathways?
The PICO framework (Population, Intervention, Comparison, Outcome) applies:
- Population : this compound in CDC reactions.
- Intervention : Varying catalysts (e.g., FeCl₃ vs. Pd(OAc)₂).
- Comparison : Non-chlorinated isoquinoline controls.
- Outcome : Regioselectivity ratios and kinetic profiles .
Q. Advanced Characterization
Q. What advanced spectroscopic techniques elucidate the electronic effects of the 8-chloro substituent?
- DFT calculations : Map electron density distribution to predict reactive sites.
- X-ray crystallography : Resolve steric effects in crystal lattices (e.g., Cl···π interactions).
- UV-Vis spectroscopy : Monitor charge-transfer transitions in metal complexes. Correlate with Hammett parameters to quantify electronic contributions .
Properties
IUPAC Name |
8-chloroisoquinoline | |
---|---|---|
Source | PubChem | |
URL | https://pubchem.ncbi.nlm.nih.gov | |
Description | Data deposited in or computed by PubChem | |
InChI |
InChI=1S/C9H6ClN/c10-9-3-1-2-7-4-5-11-6-8(7)9/h1-6H | |
Source | PubChem | |
URL | https://pubchem.ncbi.nlm.nih.gov | |
Description | Data deposited in or computed by PubChem | |
InChI Key |
OXAMVYYZTULFIB-UHFFFAOYSA-N | |
Source | PubChem | |
URL | https://pubchem.ncbi.nlm.nih.gov | |
Description | Data deposited in or computed by PubChem | |
Canonical SMILES |
C1=CC2=C(C=NC=C2)C(=C1)Cl | |
Source | PubChem | |
URL | https://pubchem.ncbi.nlm.nih.gov | |
Description | Data deposited in or computed by PubChem | |
Molecular Formula |
C9H6ClN | |
Source | PubChem | |
URL | https://pubchem.ncbi.nlm.nih.gov | |
Description | Data deposited in or computed by PubChem | |
DSSTOX Substance ID |
DTXSID70348825 | |
Record name | 8-chloroisoquinoline | |
Source | EPA DSSTox | |
URL | https://comptox.epa.gov/dashboard/DTXSID70348825 | |
Description | DSSTox provides a high quality public chemistry resource for supporting improved predictive toxicology. | |
Molecular Weight |
163.60 g/mol | |
Source | PubChem | |
URL | https://pubchem.ncbi.nlm.nih.gov | |
Description | Data deposited in or computed by PubChem | |
CAS No. |
34784-07-1 | |
Record name | 8-chloroisoquinoline | |
Source | EPA DSSTox | |
URL | https://comptox.epa.gov/dashboard/DTXSID70348825 | |
Description | DSSTox provides a high quality public chemistry resource for supporting improved predictive toxicology. | |
Synthesis routes and methods
Procedure details
Retrosynthesis Analysis
AI-Powered Synthesis Planning: Our tool employs the Template_relevance Pistachio, Template_relevance Bkms_metabolic, Template_relevance Pistachio_ringbreaker, Template_relevance Reaxys, Template_relevance Reaxys_biocatalysis model, leveraging a vast database of chemical reactions to predict feasible synthetic routes.
One-Step Synthesis Focus: Specifically designed for one-step synthesis, it provides concise and direct routes for your target compounds, streamlining the synthesis process.
Accurate Predictions: Utilizing the extensive PISTACHIO, BKMS_METABOLIC, PISTACHIO_RINGBREAKER, REAXYS, REAXYS_BIOCATALYSIS database, our tool offers high-accuracy predictions, reflecting the latest in chemical research and data.
Strategy Settings
Precursor scoring | Relevance Heuristic |
---|---|
Min. plausibility | 0.01 |
Model | Template_relevance |
Template Set | Pistachio/Bkms_metabolic/Pistachio_ringbreaker/Reaxys/Reaxys_biocatalysis |
Top-N result to add to graph | 6 |
Feasible Synthetic Routes
Disclaimer and Information on In-Vitro Research Products
Please be aware that all articles and product information presented on BenchChem are intended solely for informational purposes. The products available for purchase on BenchChem are specifically designed for in-vitro studies, which are conducted outside of living organisms. In-vitro studies, derived from the Latin term "in glass," involve experiments performed in controlled laboratory settings using cells or tissues. It is important to note that these products are not categorized as medicines or drugs, and they have not received approval from the FDA for the prevention, treatment, or cure of any medical condition, ailment, or disease. We must emphasize that any form of bodily introduction of these products into humans or animals is strictly prohibited by law. It is essential to adhere to these guidelines to ensure compliance with legal and ethical standards in research and experimentation.