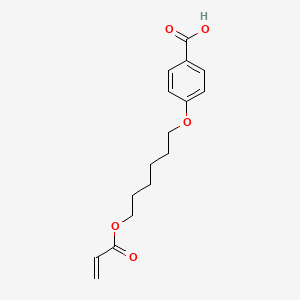
4-((6-(Acryloyloxy)hexyl)oxy)benzoic acid
Overview
Description
4-((6-(Acryloyloxy)hexyl)oxy)benzoic acid is an organic compound with the molecular formula C₁₆H₂₀O₅ and a molecular weight of 292.33 g/mol . This compound is characterized by the presence of an acryloyloxy group attached to a hexyl chain, which is further connected to a benzoic acid moiety. It is typically found as a white to yellow or pale-gray powder or crystals .
Preparation Methods
The synthesis of 4-((6-(Acryloyloxy)hexyl)oxy)benzoic acid involves the esterification of 4-(6-hydroxyhexyloxy)benzoic acid with acrylic acid. The reaction is catalyzed by p-toluenesulfonic acid and inhibited by hydroquinone to prevent polymerization . The reaction mixture is refluxed in benzene, and the water produced is removed using a Dean-Stark trap. After the reaction is complete, the solvent is distilled under reduced pressure, and the product is recrystallized from isopropyl alcohol to obtain the final compound as a white powdery solid .
Chemical Reactions Analysis
4-((6-(Acryloyloxy)hexyl)oxy)benzoic acid undergoes various chemical reactions, including:
Esterification: The compound can form esters with alcohols under acidic conditions.
Hydrolysis: The ester linkage can be hydrolyzed to yield the corresponding carboxylic acid and alcohol.
Polymerization: The acryloyloxy group can undergo free radical polymerization to form polymers.
Complexation: The carboxylate groups can form complexes with metal ions, such as copper(II), serving as bidentate ligands.
Common reagents used in these reactions include acids (e.g., hydrochloric acid for hydrolysis), bases (e.g., potassium hydroxide for saponification), and radical initiators (e.g., benzoyl peroxide for polymerization).
Scientific Research Applications
4-((6-(Acryloyloxy)hexyl)oxy)benzoic acid has several scientific research applications:
Polymer Chemistry: It is used as a monomer in the synthesis of polymers and copolymers with specific properties.
Material Science: The compound is utilized in the development of advanced materials, such as cross-linked films and coatings.
Biomedical Research: It is investigated for its potential use in drug delivery systems and biomedical devices due to its biocompatibility and functional groups.
Mechanism of Action
The mechanism of action of 4-((6-(Acryloyloxy)hexyl)oxy)benzoic acid primarily involves its ability to undergo polymerization and form cross-linked networks. The acryloyloxy group participates in free radical polymerization, leading to the formation of polymers with desired mechanical and chemical properties . Additionally, the carboxylate groups can interact with metal ions, forming stable complexes that can be used in various applications .
Comparison with Similar Compounds
4-((6-(Acryloyloxy)hexyl)oxy)benzoic acid can be compared with similar compounds such as:
4-(6-Hydroxyhexyloxy)benzoic acid: Lacks the acryloyloxy group, making it less reactive in polymerization reactions.
4-(6-Prop-2-enoyloxyhexoxy)benzoic acid: Similar structure but with a different ester group, leading to variations in reactivity and applications.
4-(6-Acryloyloxyhexyloxy)benzoic acid: Another closely related compound with similar properties and applications.
The uniqueness of this compound lies in its specific functional groups that enable diverse chemical reactions and applications in various fields.
Properties
IUPAC Name |
4-(6-prop-2-enoyloxyhexoxy)benzoic acid | |
---|---|---|
Source | PubChem | |
URL | https://pubchem.ncbi.nlm.nih.gov | |
Description | Data deposited in or computed by PubChem | |
InChI |
InChI=1S/C16H20O5/c1-2-15(17)21-12-6-4-3-5-11-20-14-9-7-13(8-10-14)16(18)19/h2,7-10H,1,3-6,11-12H2,(H,18,19) | |
Source | PubChem | |
URL | https://pubchem.ncbi.nlm.nih.gov | |
Description | Data deposited in or computed by PubChem | |
InChI Key |
FLPSQLAEXYKMGQ-UHFFFAOYSA-N | |
Source | PubChem | |
URL | https://pubchem.ncbi.nlm.nih.gov | |
Description | Data deposited in or computed by PubChem | |
Canonical SMILES |
C=CC(=O)OCCCCCCOC1=CC=C(C=C1)C(=O)O | |
Source | PubChem | |
URL | https://pubchem.ncbi.nlm.nih.gov | |
Description | Data deposited in or computed by PubChem | |
Molecular Formula |
C16H20O5 | |
Source | PubChem | |
URL | https://pubchem.ncbi.nlm.nih.gov | |
Description | Data deposited in or computed by PubChem | |
Related CAS |
122408-80-4 | |
Record name | Benzoic acid, 4-[[6-[(1-oxo-2-propen-1-yl)oxy]hexyl]oxy]-, homopolymer | |
Source | CAS Common Chemistry | |
URL | https://commonchemistry.cas.org/detail?cas_rn=122408-80-4 | |
Description | CAS Common Chemistry is an open community resource for accessing chemical information. Nearly 500,000 chemical substances from CAS REGISTRY cover areas of community interest, including common and frequently regulated chemicals, and those relevant to high school and undergraduate chemistry classes. This chemical information, curated by our expert scientists, is provided in alignment with our mission as a division of the American Chemical Society. | |
Explanation | The data from CAS Common Chemistry is provided under a CC-BY-NC 4.0 license, unless otherwise stated. | |
DSSTOX Substance ID |
DTXSID30395395 | |
Record name | 4-((6-(Acryloyloxy)hexyl)oxy)benzoic acid | |
Source | EPA DSSTox | |
URL | https://comptox.epa.gov/dashboard/DTXSID30395395 | |
Description | DSSTox provides a high quality public chemistry resource for supporting improved predictive toxicology. | |
Molecular Weight |
292.33 g/mol | |
Source | PubChem | |
URL | https://pubchem.ncbi.nlm.nih.gov | |
Description | Data deposited in or computed by PubChem | |
CAS No. |
83883-26-5 | |
Record name | 4-[[6-[(1-Oxo-2-propen-1-yl)oxy]hexyl]oxy]benzoic acid | |
Source | CAS Common Chemistry | |
URL | https://commonchemistry.cas.org/detail?cas_rn=83883-26-5 | |
Description | CAS Common Chemistry is an open community resource for accessing chemical information. Nearly 500,000 chemical substances from CAS REGISTRY cover areas of community interest, including common and frequently regulated chemicals, and those relevant to high school and undergraduate chemistry classes. This chemical information, curated by our expert scientists, is provided in alignment with our mission as a division of the American Chemical Society. | |
Explanation | The data from CAS Common Chemistry is provided under a CC-BY-NC 4.0 license, unless otherwise stated. | |
Record name | 4-((6-(Acryloyloxy)hexyl)oxy)benzoic acid | |
Source | EPA DSSTox | |
URL | https://comptox.epa.gov/dashboard/DTXSID30395395 | |
Description | DSSTox provides a high quality public chemistry resource for supporting improved predictive toxicology. | |
Synthesis routes and methods I
Procedure details
Synthesis routes and methods II
Procedure details
Synthesis routes and methods III
Procedure details
Synthesis routes and methods IV
Procedure details
Retrosynthesis Analysis
AI-Powered Synthesis Planning: Our tool employs the Template_relevance Pistachio, Template_relevance Bkms_metabolic, Template_relevance Pistachio_ringbreaker, Template_relevance Reaxys, Template_relevance Reaxys_biocatalysis model, leveraging a vast database of chemical reactions to predict feasible synthetic routes.
One-Step Synthesis Focus: Specifically designed for one-step synthesis, it provides concise and direct routes for your target compounds, streamlining the synthesis process.
Accurate Predictions: Utilizing the extensive PISTACHIO, BKMS_METABOLIC, PISTACHIO_RINGBREAKER, REAXYS, REAXYS_BIOCATALYSIS database, our tool offers high-accuracy predictions, reflecting the latest in chemical research and data.
Strategy Settings
Precursor scoring | Relevance Heuristic |
---|---|
Min. plausibility | 0.01 |
Model | Template_relevance |
Template Set | Pistachio/Bkms_metabolic/Pistachio_ringbreaker/Reaxys/Reaxys_biocatalysis |
Top-N result to add to graph | 6 |
Feasible Synthetic Routes
Q1: How does the structure of 4-((6-(acryloyloxy)hexyl)oxy)benzoic acid influence its liquid crystal properties?
A: this compound exhibits liquid crystal behavior due to its unique molecular structure. The molecule possesses a rigid, rod-like shape with a benzene ring core. This core provides structural rigidity, while the long alkyl chain and the acryloyloxy group contribute to the molecule's ability to align in a specific orientation, forming a liquid crystal phase. [, ]
Q2: How does this compound interact with lithium ions in comb-shaped liquid-crystalline ionomers, and what are the observed effects?
A: In a study on lithium-containing comb-shaped liquid-crystalline ionomers, this compound was incorporated into a butyl acrylate copolymer. [] Researchers found that increasing the concentration of lithium ions within these ionomers significantly impacted the segmental mobility of the polymer chains. This was observed as a lengthening of the relaxation times for the α process, indicating that the lithium ions interact with the this compound moieties and influence the overall chain dynamics of the ionomer. []
Q3: How does the crystalline nature of this compound contribute to its application in perovskite solar cells?
A: Research has shown that this compound acts as a printable and crystalline template for perovskite films in solar cell applications. [] The compound's liquid crystal temperature range aligns with the perovskite film's annealing temperature, ensuring printability and homogeneity. During annealing, the crystallization of this compound promotes perovskite film crystallization, improving efficiency and stress release within the layer. []
Disclaimer and Information on In-Vitro Research Products
Please be aware that all articles and product information presented on BenchChem are intended solely for informational purposes. The products available for purchase on BenchChem are specifically designed for in-vitro studies, which are conducted outside of living organisms. In-vitro studies, derived from the Latin term "in glass," involve experiments performed in controlled laboratory settings using cells or tissues. It is important to note that these products are not categorized as medicines or drugs, and they have not received approval from the FDA for the prevention, treatment, or cure of any medical condition, ailment, or disease. We must emphasize that any form of bodily introduction of these products into humans or animals is strictly prohibited by law. It is essential to adhere to these guidelines to ensure compliance with legal and ethical standards in research and experimentation.