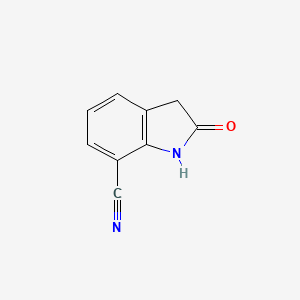
7-Cyanooxindole
Overview
Description
7-Cyanooxindole (CAS 380427-40-7) is an oxindole derivative characterized by a cyano (-CN) substituent at the 7-position of the indole ring. Its molecular formula is C₉H₆N₂O, with a molecular weight of 158.16 g/mol . Oxindoles are bicyclic compounds consisting of a benzene ring fused to a pyrrolidone ring, and the introduction of a cyano group at the 7-position enhances its polarity and reactivity, making it valuable in pharmaceutical and organic synthesis. Safety data indicate that this compound is harmful upon inhalation, skin contact, or ingestion, requiring careful handling .
Preparation Methods
Synthetic Routes and Reaction Conditions: 7-Cyanooxindole can be synthesized through several methods. One common approach involves the cyanocarbonation and hydrogenation of 7-formyl indole. This method efficiently produces this compound in good yields and can be scaled up for larger quantities, making it an attractive method for research purposes.
Industrial Production Methods: While specific industrial production methods for this compound are not extensively documented, the scalability of the cyanocarbonation/hydrogenation process suggests its potential for industrial applications. The process involves the use of appropriate catalysts and controlled reaction conditions to ensure high yield and purity.
Chemical Reactions Analysis
Types of Reactions: 7-Cyanooxindole undergoes various chemical reactions, including:
Oxidation: The compound can be oxidized under specific conditions to form different oxindole derivatives.
Reduction: Reduction reactions can convert the cyano group to other functional groups, such as amines.
Substitution: The cyano group can be substituted with other functional groups through nucleophilic substitution reactions.
Common Reagents and Conditions:
Oxidation: Common oxidizing agents include potassium permanganate and chromium trioxide.
Reduction: Reducing agents such as lithium aluminum hydride or hydrogen gas in the presence of a catalyst are typically used.
Substitution: Nucleophiles like amines or alcohols can be used in substitution reactions.
Major Products: The major products formed from these reactions depend on the specific reagents and conditions used. For example, oxidation may yield various oxindole derivatives, while reduction can produce amines or other functionalized compounds.
Scientific Research Applications
Synthesis of 7-Cyanooxindole Derivatives
The synthesis of 7-cyanooxindoles can be achieved through various methodologies, including:
- Radical-Mediated Reactions : Recent advances have shown that radical-mediated cyanoalkylation can effectively synthesize 7-cyanooxindoles using AIBN as a radical source. This method enhances the yield and purity of the desired compounds .
- Microwave-Assisted Techniques : Microwave-assisted synthesis has emerged as a powerful tool for obtaining oxindole derivatives, including this compound. This approach significantly reduces reaction times and improves yields compared to traditional heating methods .
Biological Activities
This compound exhibits a range of biological activities that make it a candidate for various therapeutic applications:
- Anticancer Properties : Several studies have indicated that derivatives of this compound possess anticancer activity. For instance, oxindole derivatives have been evaluated for their ability to inhibit cancer cell proliferation and induce apoptosis in various cancer cell lines . The mechanism often involves the modulation of key signaling pathways related to cancer progression.
- AMP-Activated Protein Kinase Inhibition : Research has identified this compound derivatives as potent inhibitors of AMP-activated protein kinase (AMPK), which plays a crucial role in cellular energy homeostasis and metabolism. These inhibitors have potential implications in treating metabolic disorders and certain types of cancer .
- Neuroprotective Effects : Some studies suggest that oxindole derivatives may exhibit neuroprotective properties, potentially offering therapeutic avenues for neurodegenerative diseases such as Alzheimer's and Parkinson's disease. The modulation of neuroinflammation and oxidative stress pathways is believed to contribute to these effects .
Case Study 1: Anticancer Activity Evaluation
A study evaluated the anticancer potential of various this compound derivatives against human cancer cell lines. The results demonstrated significant cytotoxicity, with certain compounds showing IC50 values in the low micromolar range. The study highlighted the importance of structural modifications in enhancing bioactivity .
Case Study 2: AMPK Inhibition
In a structure-activity relationship study, novel oxindole-based AMPK inhibitors were synthesized and characterized. The findings indicated that specific substitutions on the oxindole scaffold were critical for enhancing inhibitory potency against AMPK, providing insights into the design of more effective therapeutic agents .
Summary Table of Applications
Mechanism of Action
The mechanism of action of 7-Cyanooxindole and its derivatives involves their interaction with specific molecular targets and pathways. For instance, as cyclin-dependent kinase inhibitors, these compounds interfere with the cell cycle regulation by inhibiting the activity of cyclin-dependent kinases, which are crucial for cell division and proliferation. This inhibition can lead to the suppression of cancer cell growth.
Comparison with Similar Compounds
Comparison with Structurally Similar Compounds
Structural and Physicochemical Properties
The following table summarizes key differences between 7-cyanooxindole and analogous compounds:
Compound Name | CAS Number | Molecular Formula | Substituent | Molecular Weight (g/mol) | Physical State | Key Properties |
---|---|---|---|---|---|---|
This compound | 380427-40-7 | C₉H₆N₂O | -CN | 158.16 | Solid | High polarity, moderate solubility in DMSO |
7-Methyloxindole | 124700-40-9 | C₉H₉NO | -CH₃ | 147.17 | Crystalline solid | Lipophilic, low water solubility |
7-Azaindole | 271-63-6 | C₇H₆N₂ | N-atom | 118.14 | Prismatic crystals | Aromatic, basic, soluble in polar solvents |
7-Nitroindole | 6960-42-5 | C₈H₆N₂O₂ | -NO₂ | 178.15 | Yellow crystalline | Strong electron-withdrawing, reactive |
7-Methoxyindole | N/A | C₉H₉NO | -OCH₃ | 161.18 | Solid | Electron-donating, stable under acidic conditions |
Key Observations :
- Polarity: The cyano group in this compound increases polarity compared to 7-methyloxindole, enhancing solubility in polar aprotic solvents like DMSO. In contrast, 7-nitroindole’s nitro group confers even higher polarity and reactivity .
- Electronic Effects : The -CN group is electron-withdrawing, directing electrophilic substitution to the 5-position of the indole ring. This contrasts with 7-methoxyindole, where the -OCH₃ group is electron-donating, favoring substitution at the 4-position .
- Thermal Stability: 7-Azaindole’s nitrogen atom disrupts aromaticity, reducing stability compared to this compound .
This compound
The cyano group facilitates nucleophilic substitution and participates in palladium-catalyzed cross-coupling reactions. For example, it serves as a precursor in synthesizing kinase inhibitors and other bioactive molecules .
7-Methyloxindole
The methyl group is inert in most reactions, making 7-methyloxindole a poor candidate for functionalization. It is primarily used as a scaffold in natural product synthesis .
7-Azaindole
The nitrogen atom enables hydrogen bonding and coordination with metals, making it valuable in catalytic systems. It is a key intermediate in synthesizing antiviral and anticancer agents .
7-Nitroindole
The nitro group undergoes reduction to amines or serves as a directing group in electrophilic substitution. It is utilized in synthesizing fungicides and DNA-intercalating agents .
Biological Activity
7-Cyanooxindole is a synthetic compound derived from oxindole, recognized for its diverse biological activities. This article delves into the biological properties of this compound, highlighting its pharmacological potential, mechanisms of action, and relevant case studies that illustrate its therapeutic applications.
Chemical Structure
The chemical structure of this compound is characterized by the presence of a cyano group (-C≡N) at the 7-position of the oxindole framework. This modification enhances its biological activity compared to other oxindole derivatives.
Antimicrobial Activity
Research indicates that this compound exhibits significant antimicrobial properties . A study evaluated its effectiveness against various bacterial strains, revealing a minimum inhibitory concentration (MIC) as low as 6.72 mg/mL against Escherichia coli and 6.63 mg/mL against Staphylococcus aureus . The compound's mechanism involves disrupting bacterial cell wall synthesis and inhibiting essential metabolic pathways.
Anticancer Properties
This compound has shown promising anticancer activity in several studies. It induces apoptosis in cancer cells through the activation of caspases and modulation of Bcl-2 family proteins. For instance, it was found to significantly reduce cell viability in human myeloblastic leukemia cells (HL-60) . The compound's ability to inhibit tumor growth has been attributed to its interference with cell cycle progression and induction of oxidative stress.
Anti-inflammatory Effects
The compound also exhibits notable anti-inflammatory effects . In vitro studies demonstrated that it reduces the production of pro-inflammatory cytokines such as TNF-α and IL-6 in activated macrophages . This action suggests potential therapeutic applications in treating inflammatory diseases.
The biological activities of this compound are primarily mediated through:
- Caspase Activation : Inducing apoptosis in cancer cells.
- Cytokine Modulation : Decreasing pro-inflammatory cytokine levels.
- Antimicrobial Mechanisms : Disrupting bacterial cell wall integrity and metabolic functions.
Case Studies
Q & A
Basic Research Questions
Q. What are the established synthetic routes for 7-cyanooxindole, and how can researchers optimize yield and purity?
- Methodological Answer: Begin with a literature review to identify common synthetic pathways (e.g., Pd-catalyzed cyanation or radical-mediated reactions). Optimize reaction conditions (temperature, solvent, catalyst loading) using design-of-experiment (DoE) approaches to assess parameter interactions. Monitor purity via HPLC or GC-MS and validate intermediates with -/-NMR spectroscopy. Ensure reproducibility by documenting detailed protocols, including inert atmosphere requirements and quenching steps .
Q. How should researchers characterize this compound to confirm structural identity and purity?
- Methodological Answer: Combine spectroscopic techniques: -NMR for functional group analysis, -NMR to confirm cyano substitution at position 7, and IR spectroscopy for C≡N stretching (~2200 cm). Assess purity via melting point analysis and chromatographic methods (HPLC with UV detection). For novel derivatives, high-resolution mass spectrometry (HRMS) is essential. Cross-reference data with published spectra or computational predictions (e.g., DFT simulations) .
Q. What are the critical parameters for stability testing of this compound under varying storage conditions?
- Methodological Answer: Conduct accelerated stability studies under ICH guidelines (temperature: 40°C ± 2°C, humidity: 75% ± 5%) over 1–3 months. Analyze degradation products via LC-MS and quantify stability-indicating metrics (e.g., loss of parent compound >5%). Use kinetic modeling (Arrhenius equation) to predict shelf life. Include controls for photodegradation if the compound is light-sensitive .
Advanced Research Questions
Q. How can researchers resolve contradictions in reported biological activity data for this compound derivatives?
- Methodological Answer: Perform meta-analysis of published datasets to identify variability sources (e.g., assay conditions, cell lines, or compound purity). Validate key findings through independent replication using standardized protocols (e.g., NIH’s Assay Guidance Manual). Apply statistical tools (e.g., Bland-Altman plots) to assess inter-laboratory reproducibility. Consider structural analogs to isolate pharmacophore contributions .
Q. What mechanistic insights can computational modeling provide for this compound’s reactivity or binding interactions?
- Methodological Answer: Use density functional theory (DFT) to map electron density at the cyano group and predict nucleophilic/electrophilic sites. For binding studies, employ molecular dynamics (MD) simulations with force fields (e.g., AMBER) to analyze ligand-protein interactions. Validate predictions with experimental data (e.g., X-ray crystallography or isothermal titration calorimetry). Address discrepancies by refining solvation models or entropy corrections .
Q. What experimental designs are optimal for studying this compound’s role in multi-step catalytic cycles?
- Methodological Answer: Employ in situ spectroscopic monitoring (e.g., ReactIR or UV-vis) to track intermediate formation. Use isotopic labeling (e.g., -CN) to elucidate mechanistic pathways. Design control experiments to rule out non-catalytic pathways. For heterogeneous systems, pair kinetic studies with surface characterization (XPS, TEM) to correlate activity with catalyst morphology .
Q. How can researchers integrate this compound into complex natural product syntheses while minimizing side reactions?
- Methodological Answer: Use protecting group strategies (e.g., silyl ethers for hydroxyl groups) to shield reactive sites during cyanation. Optimize stepwise coupling reactions (e.g., Suzuki-Miyaura) with orthogonal reactivity. Monitor selectivity via competition experiments and adjust steric/electronic parameters of ligands or substrates. Document side products with LC-MS and refine pathways iteratively .
Q. Methodological Guidance for Data Analysis
Q. What statistical approaches are recommended for analyzing dose-response relationships in this compound bioactivity studies?
- Methodological Answer: Use nonlinear regression (e.g., Hill equation) to calculate EC values. Assess goodness-of-fit with metrics like R and AIC. For high-throughput data, apply false discovery rate (FDR) correction to minimize Type I errors. Report confidence intervals and use bootstrapping for small sample sizes. Cross-validate with orthogonal assays (e.g., SPR vs. cellular assays) .
Q. How should researchers address batch-to-batch variability in this compound synthesis for reproducible in vivo studies?
- Methodological Answer: Implement quality-by-design (QbD) principles, defining critical quality attributes (CQAs) like impurity profiles and particle size. Use multivariate analysis (PCA or PLS) to correlate process parameters with outcomes. Establish acceptance criteria for raw materials (e.g., solvent residual limits). For in vivo work, pre-test batches in pilot cohorts to confirm bioequivalence .
Q. What strategies ensure rigorous peer review of this compound research, particularly for novel methodologies?
- Methodological Answer: Pre-register studies on platforms like OSF to enhance transparency. Share raw data and code repositories (e.g., GitHub) for computational work. Use reporting standards (e.g., MIASE for enzyme kinetics, ARRIVE for animal studies). Address reviewer critiques by revising hypotheses or expanding controls, avoiding overinterpretation of preliminary data .
Properties
IUPAC Name |
2-oxo-1,3-dihydroindole-7-carbonitrile | |
---|---|---|
Source | PubChem | |
URL | https://pubchem.ncbi.nlm.nih.gov | |
Description | Data deposited in or computed by PubChem | |
InChI |
InChI=1S/C9H6N2O/c10-5-7-3-1-2-6-4-8(12)11-9(6)7/h1-3H,4H2,(H,11,12) | |
Source | PubChem | |
URL | https://pubchem.ncbi.nlm.nih.gov | |
Description | Data deposited in or computed by PubChem | |
InChI Key |
SYZZMPZKZBRDNH-UHFFFAOYSA-N | |
Source | PubChem | |
URL | https://pubchem.ncbi.nlm.nih.gov | |
Description | Data deposited in or computed by PubChem | |
Canonical SMILES |
C1C2=C(C(=CC=C2)C#N)NC1=O | |
Source | PubChem | |
URL | https://pubchem.ncbi.nlm.nih.gov | |
Description | Data deposited in or computed by PubChem | |
Molecular Formula |
C9H6N2O | |
Source | PubChem | |
URL | https://pubchem.ncbi.nlm.nih.gov | |
Description | Data deposited in or computed by PubChem | |
DSSTOX Substance ID |
DTXSID60378398 | |
Record name | 7-Cyanooxindole | |
Source | EPA DSSTox | |
URL | https://comptox.epa.gov/dashboard/DTXSID60378398 | |
Description | DSSTox provides a high quality public chemistry resource for supporting improved predictive toxicology. | |
Molecular Weight |
158.16 g/mol | |
Source | PubChem | |
URL | https://pubchem.ncbi.nlm.nih.gov | |
Description | Data deposited in or computed by PubChem | |
CAS No. |
380427-40-7 | |
Record name | 7-Cyanooxindole | |
Source | EPA DSSTox | |
URL | https://comptox.epa.gov/dashboard/DTXSID60378398 | |
Description | DSSTox provides a high quality public chemistry resource for supporting improved predictive toxicology. | |
Record name | 2-oxo-2,3-dihydro-1H-indole-7-carbonitrile | |
Source | European Chemicals Agency (ECHA) | |
URL | https://echa.europa.eu/information-on-chemicals | |
Description | The European Chemicals Agency (ECHA) is an agency of the European Union which is the driving force among regulatory authorities in implementing the EU's groundbreaking chemicals legislation for the benefit of human health and the environment as well as for innovation and competitiveness. | |
Explanation | Use of the information, documents and data from the ECHA website is subject to the terms and conditions of this Legal Notice, and subject to other binding limitations provided for under applicable law, the information, documents and data made available on the ECHA website may be reproduced, distributed and/or used, totally or in part, for non-commercial purposes provided that ECHA is acknowledged as the source: "Source: European Chemicals Agency, http://echa.europa.eu/". Such acknowledgement must be included in each copy of the material. ECHA permits and encourages organisations and individuals to create links to the ECHA website under the following cumulative conditions: Links can only be made to webpages that provide a link to the Legal Notice page. | |
Disclaimer and Information on In-Vitro Research Products
Please be aware that all articles and product information presented on BenchChem are intended solely for informational purposes. The products available for purchase on BenchChem are specifically designed for in-vitro studies, which are conducted outside of living organisms. In-vitro studies, derived from the Latin term "in glass," involve experiments performed in controlled laboratory settings using cells or tissues. It is important to note that these products are not categorized as medicines or drugs, and they have not received approval from the FDA for the prevention, treatment, or cure of any medical condition, ailment, or disease. We must emphasize that any form of bodily introduction of these products into humans or animals is strictly prohibited by law. It is essential to adhere to these guidelines to ensure compliance with legal and ethical standards in research and experimentation.