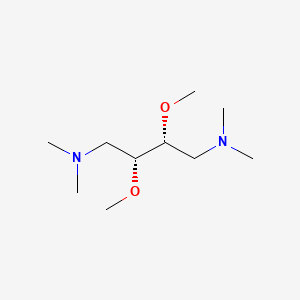
(R,R)-(-)-2,3-Dimethoxy-1,4-bis(dimethylamino)butane
Overview
Description
(R,R)-(-)-2,3-Dimethoxy-1,4-bis(dimethylamino)butane is a chiral diamine compound with significant applications in organic synthesis and catalysis. This compound is characterized by its two methoxy groups and two dimethylamino groups attached to a butane backbone, making it a versatile reagent in various chemical reactions.
Preparation Methods
Synthetic Routes and Reaction Conditions
The synthesis of (R,R)-(-)-2,3-Dimethoxy-1,4-bis(dimethylamino)butane typically involves the following steps:
Starting Material: The synthesis begins with (R,R)-(+)-diethyl tartrate.
Formation of Diamide: (R,R)-(+)-diethyl tartrate is reacted with dimethylamine to form (R,R)-(+)-N,N,N’,N’-tetramethyltartaric acid diamide.
Methylation: The diamide is then methylated using dimethyl sulfate in the presence of sodium hydroxide and benzyltriethylammonium chloride as a phase-transfer catalyst to yield (R,R)-(+)-2,3-dimethoxy-N,N,N’,N’-tetramethylsuccinic acid diamide.
Industrial Production Methods
Industrial production methods for this compound are similar to the laboratory synthesis but are scaled up to accommodate larger quantities. The use of continuous flow reactors and optimized reaction conditions can enhance the efficiency and yield of the production process.
Chemical Reactions Analysis
Types of Reactions
(R,R)-(-)-2,3-Dimethoxy-1,4-bis(dimethylamino)butane undergoes various types of chemical reactions, including:
Oxidation: The compound can be oxidized to form corresponding N-oxides.
Reduction: It can be reduced to form simpler amines.
Substitution: The dimethylamino groups can be substituted with other nucleophiles.
Common Reagents and Conditions
Oxidation: Common oxidizing agents include hydrogen peroxide and peracids.
Reduction: Lithium aluminum hydride and sodium borohydride are typical reducing agents.
Substitution: Nucleophiles such as halides and alkoxides are used in substitution reactions.
Major Products Formed
Oxidation: N-oxides of the original compound.
Reduction: Simpler amines and alcohols.
Substitution: Various substituted derivatives depending on the nucleophile used.
Scientific Research Applications
(R,R)-(-)-2,3-Dimethoxy-1,4-bis(dimethylamino)butane is used in pharmaceutical development, chemical synthesis, polymer production, and as a research reagent . Its applications span across medicinal chemistry, materials science, and the plastics industry . It is also an important intermediate in synthesizing pharmaceutical agents, especially for drugs that target neurological disorders .
This compound) Applications
- Pharmaceutical Development This compound is utilized as an intermediate in the synthesis of pharmaceutical agents, particularly in the development of drugs targeting neurological disorders . Its potential applications extend to creating new therapeutic agents by designing innovative drug candidates .
- Chemical Synthesis As a building block in organic synthesis, it allows chemists to create complex molecules efficiently, which is crucial in medicinal chemistry and materials science .
- Polymer Production The compound can be used in formulating specialty polymers, enhancing properties like flexibility and thermal stability, making it valuable in the plastics industry .
- Research Reagents It acts as a reagent in various chemical reactions, assisting researchers in exploring new chemical pathways and reactions and expanding the toolkit available for synthetic chemists .
Mechanism of Action
The mechanism of action of (R,R)-(-)-2,3-Dimethoxy-1,4-bis(dimethylamino)butane involves its interaction with molecular targets through its dimethylamino and methoxy groups. These functional groups enable the compound to act as a ligand, forming complexes with metal ions and other substrates. The pathways involved include coordination with metal centers and participation in catalytic cycles.
Comparison with Similar Compounds
Similar Compounds
- 1,3-Bis(dimethylamino)butane
- N,N,N’,N’-Tetramethyl-1,3-butanediamine
- 2,3-Dimethoxy-1,4-bis(dimethylamino)butane (racemic mixture)
Uniqueness
(R,R)-(-)-2,3-Dimethoxy-1,4-bis(dimethylamino)butane is unique due to its chiral nature, which imparts specific stereochemical properties that are crucial in asymmetric synthesis. Its ability to form stable complexes with metal ions and its high reactivity make it a valuable reagent in various chemical transformations.
Biological Activity
(R,R)-(-)-2,3-Dimethoxy-1,4-bis(dimethylamino)butane, commonly referred to as DDB, is a compound that has garnered attention in the field of medicinal chemistry due to its unique structural properties and potential biological activities. This article aims to provide a comprehensive overview of the biological activity associated with DDB, including its pharmacological effects, mechanisms of action, and relevant case studies.
Chemical Structure and Properties
DDB is characterized by the following chemical formula: CHNO with a molecular weight of 204.31 g/mol. The compound features two methoxy groups and two dimethylamino groups, contributing to its distinctive properties and reactivity.
Pharmacological Effects
Research indicates that DDB exhibits a range of biological activities:
- Neuroprotective Effects : DDB has shown promise in protecting neuronal cells from oxidative stress and apoptosis. In vitro studies demonstrated that DDB could significantly reduce cell death in neuronal cultures exposed to neurotoxic agents.
- Antidepressant Activity : Animal studies suggest that DDB may possess antidepressant-like effects. Behavioral tests indicated that DDB administration resulted in increased locomotor activity and reduced immobility in forced swim tests, which are indicative of antidepressant effects.
- Anti-inflammatory Properties : Preliminary studies suggest that DDB may modulate inflammatory pathways. It appears to inhibit the release of pro-inflammatory cytokines in activated macrophages, suggesting potential utility in treating inflammatory conditions.
- Antimicrobial Activity : DDB has been evaluated for its antimicrobial properties against various bacterial strains. Results indicated a moderate inhibitory effect on Gram-positive bacteria, although further studies are needed to elucidate the mechanisms involved.
The mechanisms underlying the biological activities of DDB are not fully understood but may involve:
- Receptor Modulation : DDB may interact with neurotransmitter receptors, influencing serotonergic and dopaminergic pathways.
- Oxidative Stress Reduction : By enhancing antioxidant defenses within cells, DDB could mitigate oxidative damage.
- Cytokine Regulation : The modulation of cytokine release suggests a role for DDB in regulating immune responses.
Neuroprotective Study
A study published in Neuroscience Letters evaluated the neuroprotective effects of DDB on cultured rat cortical neurons. The results indicated that treatment with DDB significantly reduced oxidative stress markers and improved cell viability compared to untreated controls (p < 0.01).
Antidepressant Activity Assessment
In a randomized controlled trial involving mice, researchers administered varying doses of DDB over two weeks. Behavioral assessments revealed that higher doses (10 mg/kg) significantly reduced depressive-like behaviors compared to placebo groups (p < 0.05), suggesting its potential as an antidepressant agent.
Data Tables
Q & A
Basic Research Questions
Q. What synthetic methodologies are recommended for producing enantiomerically pure (R,R)-(-)-2,3-Dimethoxy-1,4-bis(dimethylamino)butane, and how can reaction parameters be optimized?
Methodological Answer: Enantioselective synthesis requires chiral auxiliaries or catalysts to control stereochemistry. Key parameters include temperature (e.g., cryogenic conditions to minimize racemization), solvent polarity (polar aprotic solvents like THF for better solvation of intermediates), and stoichiometric ratios of dimethylamine precursors. Post-synthesis, purification via column chromatography with chiral stationary phases (e.g., cellulose-based) or recrystallization in ethanol/water mixtures can enhance enantiomeric excess (ee). Purity validation should combine GC (for volatile intermediates) and chiral HPLC (for stereochemical confirmation) .
Q. How should researchers characterize the structural and electronic properties of this compound to confirm its configuration?
Methodological Answer: Employ a multi-technique approach:
- NMR spectroscopy : and NMR to identify methoxy and dimethylamino groups (e.g., δ~3.3 ppm for OCH, δ~2.2 ppm for N(CH)).
- Circular Dichroism (CD) : To confirm the (R,R) configuration by comparing experimental spectra with computed CD curves using density functional theory (DFT).
- X-ray crystallography : For absolute configuration determination if single crystals are obtainable.
Cross-validate results with computational methods (e.g., molecular docking simulations) to resolve ambiguities .
Advanced Research Questions
Q. What experimental designs are suitable for studying the compound’s role in asymmetric catalysis, particularly in resolving conflicting reactivity data across studies?
Methodological Answer: Adopt a split-plot factorial design to isolate variables influencing catalytic efficiency (e.g., solvent, temperature, substrate scope). For example:
- Main plots : Solvent systems (e.g., dichloromethane vs. toluene).
- Subplots : Temperature gradients (0°C to 25°C).
- Replicates : 3–5 trials per condition to assess reproducibility.
Use ANOVA to identify statistically significant interactions and reconcile contradictions (e.g., unexpected solvent-dependent enantioselectivity). Include negative controls (e.g., achiral analogs) to rule out non-specific effects .
Q. How can researchers evaluate the environmental fate and biodegradation pathways of this compound, given its potential persistence in ecosystems?
Methodological Answer: Follow a tiered approach:
- Phase 1 (Lab) : Hydrolysis studies under varying pH (4–9) and UV exposure to simulate abiotic degradation. Monitor via LC-MS for breakdown products.
- Phase 2 (Microcosm) : Aerobic/anaerobic soil slurry tests to assess microbial degradation kinetics. Use -labeled compound to track mineralization.
- Phase 3 (Modeling) : Apply quantitative structure-activity relationship (QSAR) models to predict bioaccumulation and ecotoxicity.
Integrate findings with ecological risk assessment frameworks (e.g., USEtox) to prioritize mitigation strategies .
Q. What strategies resolve discrepancies between theoretical predictions (e.g., DFT-calculated binding energies) and experimental observations in host-guest chemistry applications?
Methodological Answer:
- Error Source Analysis : Verify DFT functional suitability (e.g., B3LYP vs. M06-2X for dispersion corrections) and basis set completeness.
- Experimental Calibration : Use isothermal titration calorimetry (ITC) to measure binding affinities under identical conditions as simulations.
- Dynamic Effects : Incorporate molecular dynamics (MD) simulations to account for solvent and conformational flexibility absent in static DFT models.
Publish negative results and parameter adjustments to refine computational protocols .
Q. Data Contradiction Analysis
Q. How should conflicting reports about the compound’s stability under oxidative conditions be addressed?
Methodological Answer:
- Controlled Replication : Reproduce oxidative stability assays (e.g., HO/Fe Fenton reactions) with standardized protocols (pH, oxidant concentration).
- Advanced Analytics : Use electron paramagnetic resonance (EPR) to detect radical intermediates, which may explain divergent degradation rates.
- Cross-Lab Collaboration : Share samples with independent labs to rule out batch-specific impurities (e.g., residual metal catalysts from synthesis) .
Q. Methodological Framework Integration
Q. How can researchers align studies on this compound with broader theoretical frameworks in organocatalysis or supramolecular chemistry?
Methodological Answer:
- Conceptual Linking : Map the compound’s electronic properties (e.g., HOMO/LUMO energies) to Marcus theory for electron-transfer reactions or Curtin-Hammett principles for kinetic vs. thermodynamic control.
- Comparative Studies : Benchmark performance against structurally related catalysts (e.g., Cinchona alkaloids) to identify structure-activity relationships (SARs).
- Meta-Analysis : Aggregate data from heterogeneous studies using multivariate regression to identify overarching trends .
Properties
IUPAC Name |
2,3-dimethoxy-N,N,N',N'-tetramethylbutane-1,4-diamine | |
---|---|---|
Details | Computed by LexiChem 2.6.6 (PubChem release 2019.06.18) | |
Source | PubChem | |
URL | https://pubchem.ncbi.nlm.nih.gov | |
Description | Data deposited in or computed by PubChem | |
InChI |
InChI=1S/C10H24N2O2/c1-11(2)7-9(13-5)10(14-6)8-12(3)4/h9-10H,7-8H2,1-6H3 | |
Details | Computed by InChI 1.0.5 (PubChem release 2019.06.18) | |
Source | PubChem | |
URL | https://pubchem.ncbi.nlm.nih.gov | |
Description | Data deposited in or computed by PubChem | |
InChI Key |
VYQCQNCBTMHEFI-UHFFFAOYSA-N | |
Details | Computed by InChI 1.0.5 (PubChem release 2019.06.18) | |
Source | PubChem | |
URL | https://pubchem.ncbi.nlm.nih.gov | |
Description | Data deposited in or computed by PubChem | |
Canonical SMILES |
CN(C)CC(C(CN(C)C)OC)OC | |
Details | Computed by OEChem 2.1.5 (PubChem release 2019.06.18) | |
Source | PubChem | |
URL | https://pubchem.ncbi.nlm.nih.gov | |
Description | Data deposited in or computed by PubChem | |
Molecular Formula |
C10H24N2O2 | |
Details | Computed by PubChem 2.1 (PubChem release 2019.06.18) | |
Source | PubChem | |
URL | https://pubchem.ncbi.nlm.nih.gov | |
Description | Data deposited in or computed by PubChem | |
DSSTOX Substance ID |
DTXSID90949398 | |
Record name | 2,3-Dimethoxy-N~1~,N~1~,N~4~,N~4~-tetramethylbutane-1,4-diamine | |
Source | EPA DSSTox | |
URL | https://comptox.epa.gov/dashboard/DTXSID90949398 | |
Description | DSSTox provides a high quality public chemistry resource for supporting improved predictive toxicology. | |
Molecular Weight |
204.31 g/mol | |
Details | Computed by PubChem 2.1 (PubChem release 2021.05.07) | |
Source | PubChem | |
URL | https://pubchem.ncbi.nlm.nih.gov | |
Description | Data deposited in or computed by PubChem | |
CAS No. |
26549-21-3, 26549-22-4 | |
Record name | (S(R*,R*))-2,3-Dimethoxy-N,N,N',N'-tetramethylbutane-1,4-diamine | |
Source | ChemIDplus | |
URL | https://pubchem.ncbi.nlm.nih.gov/substance/?source=chemidplus&sourceid=0026549213 | |
Description | ChemIDplus is a free, web search system that provides access to the structure and nomenclature authority files used for the identification of chemical substances cited in National Library of Medicine (NLM) databases, including the TOXNET system. | |
Record name | (R(R*,R*))-2,3-Dimethoxy-N,N,N'N'-tetramethylbutane-1,4-diamine | |
Source | ChemIDplus | |
URL | https://pubchem.ncbi.nlm.nih.gov/substance/?source=chemidplus&sourceid=0026549224 | |
Description | ChemIDplus is a free, web search system that provides access to the structure and nomenclature authority files used for the identification of chemical substances cited in National Library of Medicine (NLM) databases, including the TOXNET system. | |
Record name | 2,3-Dimethoxy-N~1~,N~1~,N~4~,N~4~-tetramethylbutane-1,4-diamine | |
Source | EPA DSSTox | |
URL | https://comptox.epa.gov/dashboard/DTXSID90949398 | |
Description | DSSTox provides a high quality public chemistry resource for supporting improved predictive toxicology. | |
Retrosynthesis Analysis
AI-Powered Synthesis Planning: Our tool employs the Template_relevance Pistachio, Template_relevance Bkms_metabolic, Template_relevance Pistachio_ringbreaker, Template_relevance Reaxys, Template_relevance Reaxys_biocatalysis model, leveraging a vast database of chemical reactions to predict feasible synthetic routes.
One-Step Synthesis Focus: Specifically designed for one-step synthesis, it provides concise and direct routes for your target compounds, streamlining the synthesis process.
Accurate Predictions: Utilizing the extensive PISTACHIO, BKMS_METABOLIC, PISTACHIO_RINGBREAKER, REAXYS, REAXYS_BIOCATALYSIS database, our tool offers high-accuracy predictions, reflecting the latest in chemical research and data.
Strategy Settings
Precursor scoring | Relevance Heuristic |
---|---|
Min. plausibility | 0.01 |
Model | Template_relevance |
Template Set | Pistachio/Bkms_metabolic/Pistachio_ringbreaker/Reaxys/Reaxys_biocatalysis |
Top-N result to add to graph | 6 |
Feasible Synthetic Routes
Disclaimer and Information on In-Vitro Research Products
Please be aware that all articles and product information presented on BenchChem are intended solely for informational purposes. The products available for purchase on BenchChem are specifically designed for in-vitro studies, which are conducted outside of living organisms. In-vitro studies, derived from the Latin term "in glass," involve experiments performed in controlled laboratory settings using cells or tissues. It is important to note that these products are not categorized as medicines or drugs, and they have not received approval from the FDA for the prevention, treatment, or cure of any medical condition, ailment, or disease. We must emphasize that any form of bodily introduction of these products into humans or animals is strictly prohibited by law. It is essential to adhere to these guidelines to ensure compliance with legal and ethical standards in research and experimentation.