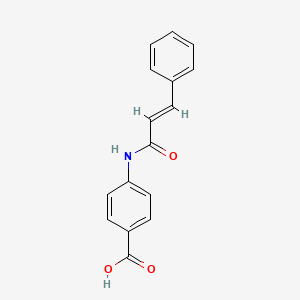
4-Cinnamamidobenzoic acid
Overview
Description
4-Cinnamamidobenzoic acid is an organic compound with the chemical formula C16H13NO3 . It has a molecular weight of 267.28 .
Synthesis Analysis
The synthesis of 4-Cinnamamidobenzoic acid involves three synthesis methods . The reaction conditions involve the use of pyridine in toluene for 12 hours .Molecular Structure Analysis
The molecular structure of 4-Cinnamamidobenzoic acid is represented by the linear formula C16H13NO3 . The InChI code for the compound is 1S/C16H13NO3/c18-15(11-6-12-4-2-1-3-5-12)17-14-9-7-13(8-10-14)16(19)20/h1-11H, (H,17,18)(H,19,20)/b11-6+ .Physical And Chemical Properties Analysis
4-Cinnamamidobenzoic acid is a solid at room temperature . It should be stored in a dry room at normal temperature .Scientific Research Applications
Antimicrobial Activity
4-Cinnamamidobenzoic acid, being a derivative of 4-aminobenzoic acid, may exhibit antimicrobial properties . The study of 4-aminobenzoic acid derivatives has shown that these compounds can be converted into antimicrobial agents . This suggests that 4-Cinnamamidobenzoic acid could potentially be used in the development of new antimicrobial drugs.
Cytotoxic Agents
In addition to their antimicrobial properties, 4-aminobenzoic acid derivatives have also been found to exhibit cytotoxic activities . This means that 4-Cinnamamidobenzoic acid could potentially be used in the development of new cancer treatments, as cytotoxic agents are often used to kill or inhibit the growth of cancer cells .
Antifungal Activity
Cinnamic acid derivatives, including 4-Cinnamamidobenzoic acid, have been found to exhibit antifungal activity . These compounds have been shown to inhibit the enzyme benzoate 4-hydroxylase (CYP53), which is unique to fungi . This makes 4-Cinnamamidobenzoic acid a potential candidate for the development of new antifungal drugs .
Inhibition of CYP53 Enzyme
The CYP53 enzyme family, which includes CYP53A15, is involved in the detoxification of benzoate, a key intermediate in aromatic compound metabolism in fungi . Cinnamic acid derivatives, including 4-Cinnamamidobenzoic acid, have been found to inhibit this enzyme . This suggests that 4-Cinnamamidobenzoic acid could be used in the development of drugs targeting the CYP53 enzyme family .
Safety and Hazards
The safety information for 4-Cinnamamidobenzoic acid indicates that it may be harmful if swallowed, causes skin irritation, causes serious eye irritation, and may cause respiratory irritation . Precautionary measures include avoiding breathing dust/fume/gas/mist/vapors/spray, washing hands thoroughly after handling, not eating, drinking or smoking when using this product, wearing protective gloves/protective clothing/eye protection/face protection, and more .
Relevant Papers One relevant paper discusses the preparation, characterization, and antibacterial properties of 4-aminocinnamic acid-modified cellulose fibers . This research could potentially provide insights into the applications of 4-Cinnamamidobenzoic acid in the development of antibacterial materials.
Mechanism of Action
Target of Action
The primary target of 4-Cinnamamidobenzoic acid is CYP53A15 , a cytochrome P450 enzyme found in the sorghum pathogen Cochliobolus lunatus . This enzyme is involved in the detoxification of benzoate, a key intermediate in aromatic compound metabolism in fungi . Because this enzyme is unique to fungi, it is a promising drug target in fungal pathogens of other eukaryotes .
Mode of Action
4-Cinnamamidobenzoic acid interacts with its target, CYP53A15, by inhibiting its enzymatic activity . This interaction disrupts the normal function of the enzyme, leading to changes in the metabolic processes of the fungi .
Biochemical Pathways
The inhibition of CYP53A15 by 4-Cinnamamidobenzoic acid affects the metabolic pathway of aromatic compounds in fungi . Specifically, it disrupts the detoxification of benzoate, a key intermediate in this pathway
Result of Action
The inhibition of CYP53A15 by 4-Cinnamamidobenzoic acid results in antifungal activity against certain fungi, including Cochliobolus lunatus, Aspergillus niger, and Pleurotus ostreatus
properties
IUPAC Name |
4-[[(E)-3-phenylprop-2-enoyl]amino]benzoic acid | |
---|---|---|
Source | PubChem | |
URL | https://pubchem.ncbi.nlm.nih.gov | |
Description | Data deposited in or computed by PubChem | |
InChI |
InChI=1S/C16H13NO3/c18-15(11-6-12-4-2-1-3-5-12)17-14-9-7-13(8-10-14)16(19)20/h1-11H,(H,17,18)(H,19,20)/b11-6+ | |
Source | PubChem | |
URL | https://pubchem.ncbi.nlm.nih.gov | |
Description | Data deposited in or computed by PubChem | |
InChI Key |
NLLZCFFEAPFEJG-IZZDOVSWSA-N | |
Source | PubChem | |
URL | https://pubchem.ncbi.nlm.nih.gov | |
Description | Data deposited in or computed by PubChem | |
Canonical SMILES |
C1=CC=C(C=C1)C=CC(=O)NC2=CC=C(C=C2)C(=O)O | |
Source | PubChem | |
URL | https://pubchem.ncbi.nlm.nih.gov | |
Description | Data deposited in or computed by PubChem | |
Isomeric SMILES |
C1=CC=C(C=C1)/C=C/C(=O)NC2=CC=C(C=C2)C(=O)O | |
Source | PubChem | |
URL | https://pubchem.ncbi.nlm.nih.gov | |
Description | Data deposited in or computed by PubChem | |
Molecular Formula |
C16H13NO3 | |
Source | PubChem | |
URL | https://pubchem.ncbi.nlm.nih.gov | |
Description | Data deposited in or computed by PubChem | |
Molecular Weight |
267.28 g/mol | |
Source | PubChem | |
URL | https://pubchem.ncbi.nlm.nih.gov | |
Description | Data deposited in or computed by PubChem | |
Product Name |
4-Cinnamamidobenzoic acid |
Retrosynthesis Analysis
AI-Powered Synthesis Planning: Our tool employs the Template_relevance Pistachio, Template_relevance Bkms_metabolic, Template_relevance Pistachio_ringbreaker, Template_relevance Reaxys, Template_relevance Reaxys_biocatalysis model, leveraging a vast database of chemical reactions to predict feasible synthetic routes.
One-Step Synthesis Focus: Specifically designed for one-step synthesis, it provides concise and direct routes for your target compounds, streamlining the synthesis process.
Accurate Predictions: Utilizing the extensive PISTACHIO, BKMS_METABOLIC, PISTACHIO_RINGBREAKER, REAXYS, REAXYS_BIOCATALYSIS database, our tool offers high-accuracy predictions, reflecting the latest in chemical research and data.
Strategy Settings
Precursor scoring | Relevance Heuristic |
---|---|
Min. plausibility | 0.01 |
Model | Template_relevance |
Template Set | Pistachio/Bkms_metabolic/Pistachio_ringbreaker/Reaxys/Reaxys_biocatalysis |
Top-N result to add to graph | 6 |
Feasible Synthetic Routes
Disclaimer and Information on In-Vitro Research Products
Please be aware that all articles and product information presented on BenchChem are intended solely for informational purposes. The products available for purchase on BenchChem are specifically designed for in-vitro studies, which are conducted outside of living organisms. In-vitro studies, derived from the Latin term "in glass," involve experiments performed in controlled laboratory settings using cells or tissues. It is important to note that these products are not categorized as medicines or drugs, and they have not received approval from the FDA for the prevention, treatment, or cure of any medical condition, ailment, or disease. We must emphasize that any form of bodily introduction of these products into humans or animals is strictly prohibited by law. It is essential to adhere to these guidelines to ensure compliance with legal and ethical standards in research and experimentation.