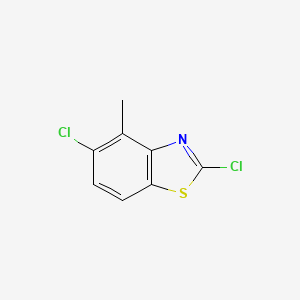
2,5-Dichloro-4-methyl-1,3-benzothiazole
- Click on QUICK INQUIRY to receive a quote from our team of experts.
- With the quality product at a COMPETITIVE price, you can focus more on your research.
Description
2,5-Dichloro-4-methyl-1,3-benzothiazole (DCMBT) is a chemical compound that belongs to the family of benzothiazoles. It is a colorless solid and can be synthesized in a laboratory. DCMBT has a wide range of applications in the field of scientific research, such as in biochemistry, physiology, and pharmacology.
Scientific Research Applications
Antitumor Activities
Antitumor Benzothiazoles Synthesis and Metabolic Studies : A series of 2-(4-aminophenyl)benzothiazoles, including derivatives of 2,5-Dichloro-4-methyl-1,3-benzothiazole, have been synthesized and investigated for their potent and selective antitumor activity against breast, ovarian, colon, and renal cell lines. The metabolism of these compounds, particularly N-acetylation and oxidation, plays a crucial role in their mechanism of action, indicating a potential novel approach to cancer therapy (Chua et al., 1999).
Chemical Synthesis and Crystal Structure
Synthesis of Benzothiazole Derivatives : Studies have shown efficient synthetic routes to various benzothiazole derivatives, including those similar to 2,5-Dichloro-4-methyl-1,3-benzothiazole. For example, bis[(E)-2-(2,4-dichloro-5-nitrostyryl)-1,3-benzothiazole] hydrate was synthesized from 2-methyl-benzothiazole, illustrating the versatility of benzothiazoles in chemical synthesis and their structural diversity (Cox et al., 1997).
Environmental Impact and Human Exposure
Benzothiazoles in the Environment and Human Exposure : Research has been conducted on the presence of benzothiazoles and their derivatives in the environment and their potential human exposure. One study focused on benzotriazoles and benzothiazoles, including compounds structurally related to 2,5-Dichloro-4-methyl-1,3-benzothiazole, in human urine from several countries to assess exposure levels. This study highlights the widespread distribution of these compounds in the environment and the need for further research into their effects on human health (Asimakopoulos et al., 2013).
properties
IUPAC Name |
2,5-dichloro-4-methyl-1,3-benzothiazole |
Source
|
---|---|---|
Source | PubChem | |
URL | https://pubchem.ncbi.nlm.nih.gov | |
Description | Data deposited in or computed by PubChem | |
InChI |
InChI=1S/C8H5Cl2NS/c1-4-5(9)2-3-6-7(4)11-8(10)12-6/h2-3H,1H3 |
Source
|
Source | PubChem | |
URL | https://pubchem.ncbi.nlm.nih.gov | |
Description | Data deposited in or computed by PubChem | |
InChI Key |
LEGBUWXKYQBBEP-UHFFFAOYSA-N |
Source
|
Source | PubChem | |
URL | https://pubchem.ncbi.nlm.nih.gov | |
Description | Data deposited in or computed by PubChem | |
Canonical SMILES |
CC1=C(C=CC2=C1N=C(S2)Cl)Cl |
Source
|
Source | PubChem | |
URL | https://pubchem.ncbi.nlm.nih.gov | |
Description | Data deposited in or computed by PubChem | |
Molecular Formula |
C8H5Cl2NS |
Source
|
Source | PubChem | |
URL | https://pubchem.ncbi.nlm.nih.gov | |
Description | Data deposited in or computed by PubChem | |
DSSTOX Substance ID |
DTXSID90365958 |
Source
|
Record name | 2,5-dichloro-4-methyl-1,3-benzothiazole | |
Source | EPA DSSTox | |
URL | https://comptox.epa.gov/dashboard/DTXSID90365958 | |
Description | DSSTox provides a high quality public chemistry resource for supporting improved predictive toxicology. | |
Molecular Weight |
218.10 g/mol |
Source
|
Source | PubChem | |
URL | https://pubchem.ncbi.nlm.nih.gov | |
Description | Data deposited in or computed by PubChem | |
Product Name |
2,5-Dichloro-4-methyl-1,3-benzothiazole | |
CAS RN |
887204-62-8 |
Source
|
Record name | 2,5-dichloro-4-methyl-1,3-benzothiazole | |
Source | EPA DSSTox | |
URL | https://comptox.epa.gov/dashboard/DTXSID90365958 | |
Description | DSSTox provides a high quality public chemistry resource for supporting improved predictive toxicology. | |
Disclaimer and Information on In-Vitro Research Products
Please be aware that all articles and product information presented on BenchChem are intended solely for informational purposes. The products available for purchase on BenchChem are specifically designed for in-vitro studies, which are conducted outside of living organisms. In-vitro studies, derived from the Latin term "in glass," involve experiments performed in controlled laboratory settings using cells or tissues. It is important to note that these products are not categorized as medicines or drugs, and they have not received approval from the FDA for the prevention, treatment, or cure of any medical condition, ailment, or disease. We must emphasize that any form of bodily introduction of these products into humans or animals is strictly prohibited by law. It is essential to adhere to these guidelines to ensure compliance with legal and ethical standards in research and experimentation.