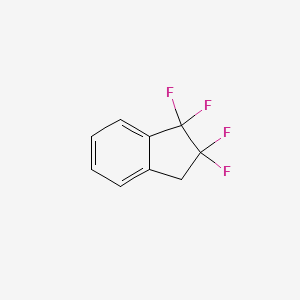
1,1,2,2-Tetrafluoro-2,3-dihydro-1H-indene
- Click on QUICK INQUIRY to receive a quote from our team of experts.
- With the quality product at a COMPETITIVE price, you can focus more on your research.
Overview
Description
1,1,2,2-Tetrafluoro-2,3-dihydro-1H-indene is a fluorinated organic compound with a unique structure that incorporates both indene and tetrafluoro groups
Preparation Methods
Synthetic Routes and Reaction Conditions
The synthesis of 1,1,2,2-tetrafluoro-2,3-dihydro-1H-indene typically involves the fluorination of indene derivatives. One common method is the reaction of indene with tetrafluoroethylene in the presence of a catalyst such as palladium or nickel. The reaction conditions often include elevated temperatures and pressures to facilitate the incorporation of fluorine atoms into the indene ring.
Industrial Production Methods
Industrial production of this compound may involve large-scale fluorination processes using specialized equipment to handle the reactive fluorine gas. The use of continuous flow reactors can enhance the efficiency and safety of the production process by providing better control over reaction conditions and minimizing the risk of hazardous by-products.
Chemical Reactions Analysis
Types of Reactions
1,1,2,2-Tetrafluoro-2,3-dihydro-1H-indene can undergo various chemical reactions, including:
Oxidation: This compound can be oxidized to form fluorinated ketones or carboxylic acids.
Reduction: Reduction reactions can convert the compound into partially or fully hydrogenated derivatives.
Substitution: Nucleophilic substitution reactions can replace one or more fluorine atoms with other functional groups.
Common Reagents and Conditions
Oxidation: Common oxidizing agents include potassium permanganate (KMnO₄) and chromium trioxide (CrO₃).
Reduction: Reducing agents such as lithium aluminum hydride (LiAlH₄) or hydrogen gas (H₂) in the presence of a metal catalyst are often used.
Substitution: Nucleophiles like amines, thiols, or alkoxides can be used under basic conditions to achieve substitution reactions.
Major Products Formed
The major products formed from these reactions depend on the specific reagents and conditions used. For example, oxidation can yield fluorinated ketones, while reduction can produce hydrogenated indene derivatives.
Scientific Research Applications
1,1,2,2-Tetrafluoro-2,3-dihydro-1H-indene has several scientific research applications:
Chemistry: It is used as a building block in the synthesis of more complex fluorinated organic molecules.
Biology: The compound’s unique fluorinated structure makes it a valuable probe in studying enzyme interactions and metabolic pathways.
Medicine: Fluorinated compounds are often explored for their potential as pharmaceuticals due to their enhanced metabolic stability and bioavailability.
Industry: It is used in the development of advanced materials, such as fluorinated polymers and coatings, which exhibit improved chemical resistance and thermal stability.
Mechanism of Action
The mechanism by which 1,1,2,2-tetrafluoro-2,3-dihydro-1H-indene exerts its effects involves interactions with molecular targets such as enzymes or receptors. The fluorine atoms in the compound can form strong hydrogen bonds and electrostatic interactions with biological molecules, influencing their activity and stability. The specific pathways involved depend on the context of its application, whether in catalysis, biological systems, or material science.
Comparison with Similar Compounds
Similar Compounds
1,1,2,2-Tetrafluoroethane: A fluorinated hydrocarbon used as a refrigerant.
2,2,3,3-Tetrafluoro-1-propanol: A fluorinated alcohol used as a solvent and intermediate in organic synthesis.
1,1,4,5-Tetramethylindane: A methylated indene derivative with different chemical properties.
Uniqueness
1,1,2,2-Tetrafluoro-2,3-dihydro-1H-indene is unique due to its combination of a fluorinated indene structure, which imparts distinct chemical and physical properties. The presence of multiple fluorine atoms enhances its stability and reactivity, making it a versatile compound for various applications in research and industry.
Biological Activity
1,1,2,2-Tetrafluoro-2,3-dihydro-1H-indene is a fluorinated organic compound that has garnered attention in various fields including medicinal chemistry and material science due to its unique structural characteristics. The presence of multiple fluorine atoms significantly impacts its biological activity, reactivity, and potential applications. This article aims to provide a comprehensive overview of the biological activity of this compound, supported by data tables and relevant research findings.
Chemical Structure and Properties
The molecular formula of this compound is C9H6F4. The compound features a bicyclic structure with four fluorine atoms attached to the indene framework. This configuration enhances its stability and alters its interaction with biological systems.
The biological activity of this compound is primarily influenced by its ability to interact with various biomolecules. The fluorine atoms contribute to increased lipophilicity and can modulate the binding affinity to molecular targets. This compound has been explored for its potential as a pharmacological agent due to its interactions with key biological pathways.
Anticancer Properties
Research indicates that certain derivatives of tetrafluoro compounds may exhibit anticancer properties by inhibiting specific pathways involved in tumor growth. For example, some studies have shown that tetrahydro derivatives can act as inhibitors of Hypoxia Inducible Factor 2α (HIF-2α), a crucial regulator in cancer progression .
Table 1: Summary of Biological Activities
Toxicological Profile
The toxicological assessment of this compound suggests that halogenated compounds can exhibit varied toxicity profiles depending on their structure. The presence of fluorine can enhance reactivity towards biological macromolecules such as DNA and proteins, potentially leading to adverse health effects including carcinogenicity .
Table 2: Toxicological Considerations
Case Studies
Several studies have investigated the biological implications of fluorinated compounds similar to this compound. One notable study focused on the effects of halogenated indenes on cellular signaling pathways related to inflammation and cancer progression. These findings highlight the need for further exploration into the specific mechanisms by which these compounds exert their biological effects.
Properties
CAS No. |
80829-35-2 |
---|---|
Molecular Formula |
C9H6F4 |
Molecular Weight |
190.14 g/mol |
IUPAC Name |
2,2,3,3-tetrafluoro-1H-indene |
InChI |
InChI=1S/C9H6F4/c10-8(11)5-6-3-1-2-4-7(6)9(8,12)13/h1-4H,5H2 |
InChI Key |
GIDWXECEYXEINM-UHFFFAOYSA-N |
Canonical SMILES |
C1C2=CC=CC=C2C(C1(F)F)(F)F |
Origin of Product |
United States |
Disclaimer and Information on In-Vitro Research Products
Please be aware that all articles and product information presented on BenchChem are intended solely for informational purposes. The products available for purchase on BenchChem are specifically designed for in-vitro studies, which are conducted outside of living organisms. In-vitro studies, derived from the Latin term "in glass," involve experiments performed in controlled laboratory settings using cells or tissues. It is important to note that these products are not categorized as medicines or drugs, and they have not received approval from the FDA for the prevention, treatment, or cure of any medical condition, ailment, or disease. We must emphasize that any form of bodily introduction of these products into humans or animals is strictly prohibited by law. It is essential to adhere to these guidelines to ensure compliance with legal and ethical standards in research and experimentation.