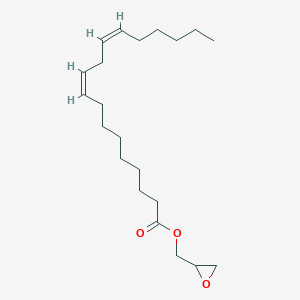
Glycidyl Linoleate
Overview
Description
Linoleic acid glycidyl ester is an esterified form of linoleic acid containing a glycidyl group. It is a compound of interest due to its presence in various processed oils and foodstuffs, including some baby formulas . The compound is hydrolyzed in vivo to glycidol, which is known to be carcinogenic in rats .
Mechanism of Action
Target of Action
Glycidyl Linoleate is a type of glycidyl ester, which are formed at high temperatures during edible oil production . The primary targets of this compound are a variety of fatty acids, such as glycidyl esters and 3-MCPD diesters of palmitate (C16:0), stearate (C18:0), oleate (C18:1), linoleate (C18:2), and linolenate (C18:3) . These fatty acids play crucial roles in various biological processes.
Mode of Action
When ingested as part of the diet, under the action of lipases, this compound and other glycidyl esters are believed to release glycidol and 3-MCPD . Glycidol is an epoxide, which can react with a wide range of biological molecules, potentially leading to various biological effects .
Biochemical Pathways
It is known that unsaturated fatty acids like linoleic acid (c18:2) play multiple crucial roles in plants and are also important economic traits of oil crops . They serve as intrinsic antioxidants, precursors of various bioactive molecules, and stocks of extracellular barrier constituents .
Pharmacokinetics
It is known that glycidyl fatty acid esters could in theory be hydrolysed by lipases to the parent glycidol in the gastrointestinal tract . This suggests that the bioavailability of this compound may be influenced by factors such as diet and the presence of specific enzymes in the body.
Result of Action
It is known that glycidol, the compound released from this compound by the action of lipases, is an epoxide with a group 2a designation by iarc—probably carcinogenic to humans .
Action Environment
The formation of this compound and other glycidyl esters may be affected by food ingredients, cooking device, and heating time, as well as heating temperature . For example, when cookie and cupcake doughs prepared with rapeseed oil were heated in the oven, glycidyl esters were formed in both samples, reaching a maximum at 210 °C .
Biochemical Analysis
Biochemical Properties
Glycidyl Linoleate is involved in various biochemical reactions. It is believed to be hydrolyzed by lipases to the parent glycidol in the gastrointestinal tract . Glycidol is an epoxide with a Group 2A designation by IARC—probably carcinogenic to humans .
Cellular Effects
The effects of this compound on cells are complex and multifaceted. For instance, linoleate, a member of the n-6 family, has been shown to significantly suppress the production of the proinflammatory cytokine interleukin-8 (IL8) in hepatocytes
Molecular Mechanism
The molecular mechanism of this compound involves its conversion to glycidol. Glycidol is a genotoxic carcinogen, and it is believed that the majority of glycidol is bound to a variety of fatty acids, such as glycidyl esters . When ingested as part of the diet, under the action of lipases, this compound is believed to release glycidol .
Temporal Effects in Laboratory Settings
In laboratory settings, the effects of this compound can change over time. For instance, it has been observed that the formation of glycidyl fatty acid esters and 3-monochloro-1,2-propanediol fatty acid esters may be affected by food ingredients, cooking device, and heating time, as well as heating temperature .
Metabolic Pathways
This compound is involved in the metabolic pathway of glycidol formation. Glycidol is formed during the deodorization process at high temperatures in the refining process of edible oil
Preparation Methods
Synthetic Routes and Reaction Conditions: Linoleic acid glycidyl ester can be synthesized through the esterification of linoleic acid with glycidol. The reaction typically involves the use of a catalyst and is carried out under controlled temperature and pressure conditions to ensure the formation of the ester bond .
Industrial Production Methods: In industrial settings, the production of glycidyl esters, including linoleic acid glycidyl ester, often occurs during the deodorization step of oil refining. This process involves heating oils at temperatures above 200°C, which leads to the formation of glycidyl esters through a series of reactions that break down fats and oils .
Chemical Reactions Analysis
Types of Reactions: Linoleic acid glycidyl ester undergoes various chemical reactions, including:
Hydrolysis: The ester bond can be hydrolyzed by lipases in the gastrointestinal tract, releasing glycidol and linoleic acid.
Substitution: The glycidyl group can participate in nucleophilic substitution reactions, where the epoxide ring is opened by nucleophiles.
Common Reagents and Conditions:
Hydrolysis: Catalyzed by lipases or acidic/basic conditions.
Oxidation: Typically occurs under ambient conditions with exposure to air and light.
Substitution: Requires nucleophiles such as amines or thiols under mild conditions.
Major Products:
Hydrolysis: Glycidol and linoleic acid.
Oxidation: Peroxides and other oxidative degradation products.
Substitution: Various substituted glycidyl derivatives depending on the nucleophile used.
Scientific Research Applications
Linoleic acid glycidyl ester has several applications in scientific research:
Comparison with Similar Compounds
Linoleic acid glycidyl ester is similar to other glycidyl esters of fatty acids, such as:
- Palmitic acid glycidyl ester
- Stearic acid glycidyl ester
- Oleic acid glycidyl ester
- Linolenic acid glycidyl ester
Uniqueness: Linoleic acid glycidyl ester is unique due to its specific fatty acid component, linoleic acid, which is an essential fatty acid with two double bonds. This structural feature influences its chemical reactivity and biological effects compared to other glycidyl esters .
Properties
IUPAC Name |
oxiran-2-ylmethyl (9Z,12Z)-octadeca-9,12-dienoate | |
---|---|---|
Source | PubChem | |
URL | https://pubchem.ncbi.nlm.nih.gov | |
Description | Data deposited in or computed by PubChem | |
InChI |
InChI=1S/C21H36O3/c1-2-3-4-5-6-7-8-9-10-11-12-13-14-15-16-17-21(22)24-19-20-18-23-20/h6-7,9-10,20H,2-5,8,11-19H2,1H3/b7-6-,10-9- | |
Source | PubChem | |
URL | https://pubchem.ncbi.nlm.nih.gov | |
Description | Data deposited in or computed by PubChem | |
InChI Key |
LOGTZDQTPQYKEN-HZJYTTRNSA-N | |
Source | PubChem | |
URL | https://pubchem.ncbi.nlm.nih.gov | |
Description | Data deposited in or computed by PubChem | |
Canonical SMILES |
CCCCCC=CCC=CCCCCCCCC(=O)OCC1CO1 | |
Source | PubChem | |
URL | https://pubchem.ncbi.nlm.nih.gov | |
Description | Data deposited in or computed by PubChem | |
Isomeric SMILES |
CCCCC/C=C\C/C=C\CCCCCCCC(=O)OCC1CO1 | |
Source | PubChem | |
URL | https://pubchem.ncbi.nlm.nih.gov | |
Description | Data deposited in or computed by PubChem | |
Molecular Formula |
C21H36O3 | |
Source | PubChem | |
URL | https://pubchem.ncbi.nlm.nih.gov | |
Description | Data deposited in or computed by PubChem | |
DSSTOX Substance ID |
DTXSID30635228 | |
Record name | (Oxiran-2-yl)methyl (9Z,12Z)-octadeca-9,12-dienoate | |
Source | EPA DSSTox | |
URL | https://comptox.epa.gov/dashboard/DTXSID30635228 | |
Description | DSSTox provides a high quality public chemistry resource for supporting improved predictive toxicology. | |
Molecular Weight |
336.5 g/mol | |
Source | PubChem | |
URL | https://pubchem.ncbi.nlm.nih.gov | |
Description | Data deposited in or computed by PubChem | |
CAS No. |
24305-63-3 | |
Record name | Glycidyl linoleate | |
Source | CAS Common Chemistry | |
URL | https://commonchemistry.cas.org/detail?cas_rn=24305-63-3 | |
Description | CAS Common Chemistry is an open community resource for accessing chemical information. Nearly 500,000 chemical substances from CAS REGISTRY cover areas of community interest, including common and frequently regulated chemicals, and those relevant to high school and undergraduate chemistry classes. This chemical information, curated by our expert scientists, is provided in alignment with our mission as a division of the American Chemical Society. | |
Explanation | The data from CAS Common Chemistry is provided under a CC-BY-NC 4.0 license, unless otherwise stated. | |
Record name | (Oxiran-2-yl)methyl (9Z,12Z)-octadeca-9,12-dienoate | |
Source | EPA DSSTox | |
URL | https://comptox.epa.gov/dashboard/DTXSID30635228 | |
Description | DSSTox provides a high quality public chemistry resource for supporting improved predictive toxicology. | |
Retrosynthesis Analysis
AI-Powered Synthesis Planning: Our tool employs the Template_relevance Pistachio, Template_relevance Bkms_metabolic, Template_relevance Pistachio_ringbreaker, Template_relevance Reaxys, Template_relevance Reaxys_biocatalysis model, leveraging a vast database of chemical reactions to predict feasible synthetic routes.
One-Step Synthesis Focus: Specifically designed for one-step synthesis, it provides concise and direct routes for your target compounds, streamlining the synthesis process.
Accurate Predictions: Utilizing the extensive PISTACHIO, BKMS_METABOLIC, PISTACHIO_RINGBREAKER, REAXYS, REAXYS_BIOCATALYSIS database, our tool offers high-accuracy predictions, reflecting the latest in chemical research and data.
Strategy Settings
Precursor scoring | Relevance Heuristic |
---|---|
Min. plausibility | 0.01 |
Model | Template_relevance |
Template Set | Pistachio/Bkms_metabolic/Pistachio_ringbreaker/Reaxys/Reaxys_biocatalysis |
Top-N result to add to graph | 6 |
Feasible Synthetic Routes
Disclaimer and Information on In-Vitro Research Products
Please be aware that all articles and product information presented on BenchChem are intended solely for informational purposes. The products available for purchase on BenchChem are specifically designed for in-vitro studies, which are conducted outside of living organisms. In-vitro studies, derived from the Latin term "in glass," involve experiments performed in controlled laboratory settings using cells or tissues. It is important to note that these products are not categorized as medicines or drugs, and they have not received approval from the FDA for the prevention, treatment, or cure of any medical condition, ailment, or disease. We must emphasize that any form of bodily introduction of these products into humans or animals is strictly prohibited by law. It is essential to adhere to these guidelines to ensure compliance with legal and ethical standards in research and experimentation.