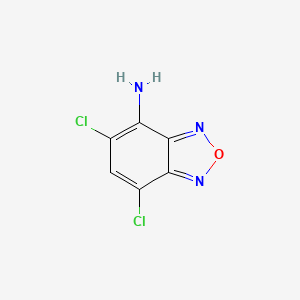
5,7-Dichloro-2,1,3-benzoxadiazol-4-amine
- Click on QUICK INQUIRY to receive a quote from our team of experts.
- With the quality product at a COMPETITIVE price, you can focus more on your research.
Overview
Description
5,7-Dichloro-2,1,3-benzoxadiazol-4-amine is a chemical compound with the CAS Number: 330982-41-7 and Linear Formula: C6H3Cl2N3O . It has a molecular weight of 204.01 .
Molecular Structure Analysis
The InChI Code for this compound is 1S/C6H3Cl2N3O/c7-2-1-3(8)5-6(4(2)9)11-12-10-5/h1H,9H2 . This code represents the molecular structure of the compound.Physical And Chemical Properties Analysis
The compound is a solid at room temperature . It should be stored in a refrigerated environment . .Scientific Research Applications
Environmental Chemistry and Toxicology
A significant application of halogenated benzoxadiazol derivatives is in understanding environmental pollution and toxicology. For instance, studies on halogenated indigo dyes have demonstrated how derivatives like 5,7-Dichloro-2,1,3-benzoxadiazol-4-amine can be sources of halogenated carbazoles in the environment. These compounds, due to their persistence and potential dioxin-like toxicological impacts, highlight the environmental implications of industrial practices that utilize halogenated aromatic compounds (Parette et al., 2015).
Synthetic Chemistry
In synthetic chemistry, the reactivity of amines, including those related to this compound, has been explored to develop novel compounds with potential therapeutic applications. Research on the synthesis of oxadiazoles reviews the methods used for the synthesis of 3,5-substituted 1,2,4-oxadiazoles, emphasizing the role of primary amidoximes and acylating agents as initial reactants. This highlights the compound's relevance in synthesizing heterocyclic compounds with diverse biological activities (Kayukova, 2005).
Medicinal Chemistry
The exploration of 1,3,4-oxadiazole derivatives, related structurally to this compound, has shown significant therapeutic potential. Compounds featuring the 1,3,4-oxadiazole ring have been identified for their binding efficiency with various enzymes and receptors, suggesting a broad spectrum of pharmacological activities. This includes anticancer, antibacterial, and antifungal properties, underscoring the importance of such derivatives in drug development and the potential for future research to expand their medicinal applications (Verma et al., 2019).
Safety and Hazards
properties
IUPAC Name |
5,7-dichloro-2,1,3-benzoxadiazol-4-amine |
Source
|
---|---|---|
Source | PubChem | |
URL | https://pubchem.ncbi.nlm.nih.gov | |
Description | Data deposited in or computed by PubChem | |
InChI |
InChI=1S/C6H3Cl2N3O/c7-2-1-3(8)5-6(4(2)9)11-12-10-5/h1H,9H2 |
Source
|
Source | PubChem | |
URL | https://pubchem.ncbi.nlm.nih.gov | |
Description | Data deposited in or computed by PubChem | |
InChI Key |
LPYPMZSBOBFGBF-UHFFFAOYSA-N |
Source
|
Source | PubChem | |
URL | https://pubchem.ncbi.nlm.nih.gov | |
Description | Data deposited in or computed by PubChem | |
Canonical SMILES |
C1=C(C2=NON=C2C(=C1Cl)N)Cl |
Source
|
Source | PubChem | |
URL | https://pubchem.ncbi.nlm.nih.gov | |
Description | Data deposited in or computed by PubChem | |
Molecular Formula |
C6H3Cl2N3O |
Source
|
Source | PubChem | |
URL | https://pubchem.ncbi.nlm.nih.gov | |
Description | Data deposited in or computed by PubChem | |
DSSTOX Substance ID |
DTXSID30346049 |
Source
|
Record name | 5,7-Dichloro-2,1,3-benzoxadiazol-4-amine | |
Source | EPA DSSTox | |
URL | https://comptox.epa.gov/dashboard/DTXSID30346049 | |
Description | DSSTox provides a high quality public chemistry resource for supporting improved predictive toxicology. | |
Molecular Weight |
204.01 g/mol |
Source
|
Source | PubChem | |
URL | https://pubchem.ncbi.nlm.nih.gov | |
Description | Data deposited in or computed by PubChem | |
CAS RN |
330982-41-7 |
Source
|
Record name | 5,7-Dichloro-2,1,3-benzoxadiazol-4-amine | |
Source | EPA DSSTox | |
URL | https://comptox.epa.gov/dashboard/DTXSID30346049 | |
Description | DSSTox provides a high quality public chemistry resource for supporting improved predictive toxicology. | |
Disclaimer and Information on In-Vitro Research Products
Please be aware that all articles and product information presented on BenchChem are intended solely for informational purposes. The products available for purchase on BenchChem are specifically designed for in-vitro studies, which are conducted outside of living organisms. In-vitro studies, derived from the Latin term "in glass," involve experiments performed in controlled laboratory settings using cells or tissues. It is important to note that these products are not categorized as medicines or drugs, and they have not received approval from the FDA for the prevention, treatment, or cure of any medical condition, ailment, or disease. We must emphasize that any form of bodily introduction of these products into humans or animals is strictly prohibited by law. It is essential to adhere to these guidelines to ensure compliance with legal and ethical standards in research and experimentation.