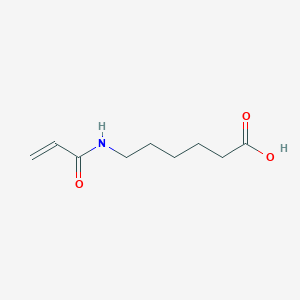
6-Acrylamidohexanoic Acid
Overview
Description
6-Acrylamidohexanoic Acid is a versatile carboxylic acid with the molecular formula C9H15NO3 and a molecular weight of 185.22 g/mol . It is a colorless, odorless, and water-soluble compound that serves as an intermediate in synthesizing a diverse range of compounds . This compound is widely used in pharmaceuticals, cosmetics, and food additives .
Preparation Methods
Synthetic Routes and Reaction Conditions: 6-Acrylamidohexanoic Acid can be synthesized through the dehydrative condensation reaction of N-hydroxysulfosuccinimide sodium salt with this compound . This reaction typically involves the use of a dehydrating agent to facilitate the formation of the desired product.
Industrial Production Methods: In industrial settings, this compound is produced using similar synthetic routes but on a larger scale. The reaction conditions are optimized to ensure high yield and purity of the final product. The compound is often purified through crystallization or other separation techniques to achieve the desired quality .
Chemical Reactions Analysis
Types of Reactions: 6-Acrylamidohexanoic Acid undergoes various chemical reactions, including:
Oxidation: The compound can be oxidized to form corresponding carboxylic acids.
Reduction: Reduction reactions can convert the compound into its corresponding alcohols.
Substitution: The amide group in this compound can undergo substitution reactions with various nucleophiles.
Common Reagents and Conditions:
Oxidation: Common oxidizing agents include potassium permanganate and chromium trioxide.
Reduction: Reducing agents such as lithium aluminum hydride and sodium borohydride are commonly used.
Substitution: Nucleophiles like amines and alcohols are used in substitution reactions.
Major Products Formed:
Oxidation: Carboxylic acids.
Reduction: Alcohols.
Substitution: Amides and esters.
Scientific Research Applications
6-Acrylamidohexanoic Acid has extensive applications in scientific research, including:
Chemistry: It is used as a monomer in polymer synthesis and as a crosslinker in polyacrylamide gels.
Biology: The compound is employed in the study of cell-extracellular matrix interactions and protein binding.
Medicine: It serves as an intermediate in the synthesis of various pharmaceuticals.
Industry: The compound is used in the production of cosmetics and food additives.
Mechanism of Action
The exact mechanism of action of 6-Acrylamidohexanoic Acid is not fully understood. it is known to act as a catalyst in compound synthesis. The compound interacts with molecular targets and pathways involved in the synthesis of peptides and proteins .
Comparison with Similar Compounds
6-Aminocaproic Acid: Used in the synthesis of 6-Acrylamidohexanoic Acid.
N-Hydroxysuccinimide: A reagent used in the synthesis of this compound.
Uniqueness: this compound is unique due to its versatility and wide range of applications in various fields. Its ability to undergo multiple chemical reactions and serve as an intermediate in the synthesis of diverse compounds makes it a valuable chemical in scientific research and industrial applications .
Properties
IUPAC Name |
6-(prop-2-enoylamino)hexanoic acid | |
---|---|---|
Source | PubChem | |
URL | https://pubchem.ncbi.nlm.nih.gov | |
Description | Data deposited in or computed by PubChem | |
InChI |
InChI=1S/C9H15NO3/c1-2-8(11)10-7-5-3-4-6-9(12)13/h2H,1,3-7H2,(H,10,11)(H,12,13) | |
Source | PubChem | |
URL | https://pubchem.ncbi.nlm.nih.gov | |
Description | Data deposited in or computed by PubChem | |
InChI Key |
SAQWCPXBLNGTCC-UHFFFAOYSA-N | |
Source | PubChem | |
URL | https://pubchem.ncbi.nlm.nih.gov | |
Description | Data deposited in or computed by PubChem | |
Canonical SMILES |
C=CC(=O)NCCCCCC(=O)O | |
Source | PubChem | |
URL | https://pubchem.ncbi.nlm.nih.gov | |
Description | Data deposited in or computed by PubChem | |
Molecular Formula |
C9H15NO3 | |
Source | PubChem | |
URL | https://pubchem.ncbi.nlm.nih.gov | |
Description | Data deposited in or computed by PubChem | |
DSSTOX Substance ID |
DTXSID60314796 | |
Record name | 6-Acrylamidohexanoic Acid | |
Source | EPA DSSTox | |
URL | https://comptox.epa.gov/dashboard/DTXSID60314796 | |
Description | DSSTox provides a high quality public chemistry resource for supporting improved predictive toxicology. | |
Molecular Weight |
185.22 g/mol | |
Source | PubChem | |
URL | https://pubchem.ncbi.nlm.nih.gov | |
Description | Data deposited in or computed by PubChem | |
CAS No. |
20766-85-2 | |
Record name | 20766-85-2 | |
Source | DTP/NCI | |
URL | https://dtp.cancer.gov/dtpstandard/servlet/dwindex?searchtype=NSC&outputformat=html&searchlist=288649 | |
Description | The NCI Development Therapeutics Program (DTP) provides services and resources to the academic and private-sector research communities worldwide to facilitate the discovery and development of new cancer therapeutic agents. | |
Explanation | Unless otherwise indicated, all text within NCI products is free of copyright and may be reused without our permission. Credit the National Cancer Institute as the source. | |
Record name | 6-Acrylamidohexanoic Acid | |
Source | EPA DSSTox | |
URL | https://comptox.epa.gov/dashboard/DTXSID60314796 | |
Description | DSSTox provides a high quality public chemistry resource for supporting improved predictive toxicology. | |
Record name | 6-Acrylamidohexanoic Acid | |
Source | European Chemicals Agency (ECHA) | |
URL | https://echa.europa.eu/information-on-chemicals | |
Description | The European Chemicals Agency (ECHA) is an agency of the European Union which is the driving force among regulatory authorities in implementing the EU's groundbreaking chemicals legislation for the benefit of human health and the environment as well as for innovation and competitiveness. | |
Explanation | Use of the information, documents and data from the ECHA website is subject to the terms and conditions of this Legal Notice, and subject to other binding limitations provided for under applicable law, the information, documents and data made available on the ECHA website may be reproduced, distributed and/or used, totally or in part, for non-commercial purposes provided that ECHA is acknowledged as the source: "Source: European Chemicals Agency, http://echa.europa.eu/". Such acknowledgement must be included in each copy of the material. ECHA permits and encourages organisations and individuals to create links to the ECHA website under the following cumulative conditions: Links can only be made to webpages that provide a link to the Legal Notice page. | |
Synthesis routes and methods I
Procedure details
Synthesis routes and methods II
Procedure details
Synthesis routes and methods III
Procedure details
Synthesis routes and methods IV
Procedure details
Retrosynthesis Analysis
AI-Powered Synthesis Planning: Our tool employs the Template_relevance Pistachio, Template_relevance Bkms_metabolic, Template_relevance Pistachio_ringbreaker, Template_relevance Reaxys, Template_relevance Reaxys_biocatalysis model, leveraging a vast database of chemical reactions to predict feasible synthetic routes.
One-Step Synthesis Focus: Specifically designed for one-step synthesis, it provides concise and direct routes for your target compounds, streamlining the synthesis process.
Accurate Predictions: Utilizing the extensive PISTACHIO, BKMS_METABOLIC, PISTACHIO_RINGBREAKER, REAXYS, REAXYS_BIOCATALYSIS database, our tool offers high-accuracy predictions, reflecting the latest in chemical research and data.
Strategy Settings
Precursor scoring | Relevance Heuristic |
---|---|
Min. plausibility | 0.01 |
Model | Template_relevance |
Template Set | Pistachio/Bkms_metabolic/Pistachio_ringbreaker/Reaxys/Reaxys_biocatalysis |
Top-N result to add to graph | 6 |
Feasible Synthetic Routes
Q1: How does the incorporation of 6-acrylamidohexanoic acid (AACA) influence the properties of polyvinyl alcohol (PVA) hydrogels?
A1: Incorporating AACA into PVA hydrogels, along with a borax cross-linker, significantly enhances their mechanical strength compared to PVA hydrogels cross-linked solely with borax [, ]. This improvement stems from the introduction of multiple physical interactions within the hydrogel network. Specifically, AACA contributes to electrostatic interactions and hydrogen bonds, supplementing the existing network structure. These additional interactions contribute to the enhanced strength observed in these modified hydrogels.
Q2: Can you explain the self-healing properties observed in PVA/AACA hydrogels and their relation to pH?
A2: PVA/AACA hydrogels exhibit the intriguing property of self-healing in both acidic and alkaline conditions []. This behavior is attributed to the dynamic nature of the non-covalent interactions, such as hydrogen bonds and electrostatic interactions, present within the hydrogel structure. In acidic conditions, the carboxylic acid groups of AACA become protonated, weakening the electrostatic interactions but potentially strengthening hydrogen bonding interactions. Conversely, in alkaline environments, deprotonation of the carboxylic acid groups strengthens electrostatic interactions. This dynamic interplay of interactions allows the hydrogel network to readily reform after disruption, leading to self-healing capabilities in both acidic and alkaline environments.
Disclaimer and Information on In-Vitro Research Products
Please be aware that all articles and product information presented on BenchChem are intended solely for informational purposes. The products available for purchase on BenchChem are specifically designed for in-vitro studies, which are conducted outside of living organisms. In-vitro studies, derived from the Latin term "in glass," involve experiments performed in controlled laboratory settings using cells or tissues. It is important to note that these products are not categorized as medicines or drugs, and they have not received approval from the FDA for the prevention, treatment, or cure of any medical condition, ailment, or disease. We must emphasize that any form of bodily introduction of these products into humans or animals is strictly prohibited by law. It is essential to adhere to these guidelines to ensure compliance with legal and ethical standards in research and experimentation.