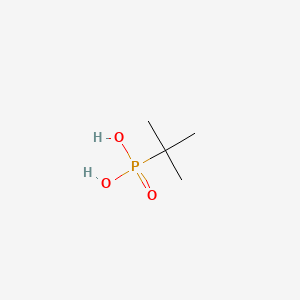
tert-Butylphosphonic acid
Overview
Description
tert-Butylphosphonic acid: is an organophosphorus compound with the chemical formula (CH₃)₃CP(O)(OH)₂. It is a phosphonic acid derivative where the phosphorus atom is bonded to a tert-butyl group. This compound is known for its stability and versatility in various chemical reactions and applications.
Mechanism of Action
Target of Action
tert-Butylphosphonic acid (t-BuPO3H2) is an organic phosphorus compound . It has been used in the preparation of various complexes, including dinuclear dicationic vanadium (IV) complexes . These complexes are the primary targets of t-BuPO3H2. The role of these complexes can vary depending on the specific application, but they often serve as catalysts in various chemical reactions .
Mode of Action
The mode of action of t-BuPO3H2 involves its interaction with its targets to form new compounds. For example, the reaction of vanadium(III) trichloride with t-BuPO3H2 in the presence of 1,10-phenanthroline/2,2’-bipyridine as an ancillary ligand in acetonitrile at room temperature afforded two dinuclear dicationic vanadium(IV) complexes . This suggests that t-BuPO3H2 can interact with its targets to form stable complexes.
Biochemical Pathways
It’s known that t-bupo3h2 can interact with nucleobases cytosine (cyt) and adenine (aden) to form hydrogen-bonded supramolecular motifs . This suggests that t-BuPO3H2 may have implications in understanding similar interactions in biological systems .
Pharmacokinetics
It’s known that t-bupo3h2 is soluble in water and many organic solvents such as alcohols, ethers, and esters . This solubility can impact the bioavailability of t-BuPO3H2, as it can be easily distributed in various environments.
Result of Action
The result of t-BuPO3H2’s action can vary depending on its specific application. For example, in the preparation of dinuclear dicationic vanadium (IV) complexes, t-BuPO3H2 helps form stable complexes that can serve as catalysts in various chemical reactions . In its interaction with nucleobases, t-BuPO3H2 forms hydrogen-bonded supramolecular motifs, which can have implications in understanding similar interactions in biological systems .
Action Environment
The action of t-BuPO3H2 can be influenced by various environmental factors. For instance, it’s known to be relatively stable under normal temperature conditions, but it may decompose under high temperature or strong oxidizing conditions . Furthermore, the presence of other chemicals, such as nucleobases or vanadium(III) trichloride, can influence the action, efficacy, and stability of t-BuPO3H2 .
Biochemical Analysis
Biochemical Properties
Tert-Butylphosphonic acid plays a significant role in biochemical reactions due to its ability to interact with enzymes, proteins, and other biomolecules. It can act as a ligand, forming complexes with metal ions that are essential cofactors for many enzymes. For example, this compound has been used in the preparation of dinuclear dicationic vanadium (IV) complexes, which are important in various catalytic processes . Additionally, this compound can interact with nucleobases such as cytosine and adenine, forming hydrogen-bonded supramolecular motifs . These interactions can influence the structure and function of nucleic acids and proteins, thereby affecting various biochemical pathways.
Cellular Effects
This compound can influence various cellular processes and functions. It has been shown to interact with cell signaling pathways, gene expression, and cellular metabolism. For instance, this compound can form complexes with nucleobases, which can affect the structure and function of DNA and RNA . This, in turn, can influence gene expression and cellular responses to environmental stimuli. Additionally, this compound can interact with enzymes involved in metabolic pathways, potentially altering cellular metabolism and energy production.
Molecular Mechanism
The molecular mechanism of action of this compound involves its ability to form stable complexes with metal ions and nucleobases. These interactions can lead to enzyme inhibition or activation, changes in gene expression, and alterations in cellular signaling pathways. For example, this compound can inhibit or activate enzymes by binding to their active sites or by forming complexes with essential cofactors . Additionally, its interaction with nucleobases can affect the structure and function of nucleic acids, leading to changes in gene expression and cellular responses .
Temporal Effects in Laboratory Settings
In laboratory settings, the effects of this compound can change over time due to its stability and degradation. This compound is relatively stable at room temperature but can degrade under high temperatures or strong oxidative conditions . Long-term studies have shown that this compound can have lasting effects on cellular function, including alterations in gene expression and metabolic pathways. These effects can be observed in both in vitro and in vivo studies, highlighting the importance of considering the temporal aspects of its use in research.
Dosage Effects in Animal Models
The effects of this compound can vary with different dosages in animal models. At low doses, it may have minimal impact on cellular function, while higher doses can lead to significant changes in gene expression, enzyme activity, and cellular metabolism. Toxic or adverse effects may be observed at very high doses, including potential damage to cellular structures and disruption of metabolic pathways . It is important to carefully consider the dosage when using this compound in animal studies to avoid potential toxicity and ensure accurate results.
Metabolic Pathways
This compound is involved in various metabolic pathways, interacting with enzymes and cofactors that are essential for cellular function. It can act as a ligand, forming complexes with metal ions that are required for enzyme activity . These interactions can influence metabolic flux and the levels of metabolites within cells. Additionally, this compound can affect the stability and function of metabolic enzymes, potentially altering the overall metabolic profile of cells.
Transport and Distribution
Within cells and tissues, this compound is transported and distributed through interactions with transporters and binding proteins. These interactions can influence its localization and accumulation within specific cellular compartments . For example, this compound can bind to metal ions and transport proteins, facilitating its movement across cellular membranes and into target tissues. Understanding the transport and distribution of this compound is crucial for determining its effects on cellular function and overall organism health.
Subcellular Localization
The subcellular localization of this compound can affect its activity and function. It may be directed to specific compartments or organelles through targeting signals or post-translational modifications . For instance, this compound can localize to the nucleus, where it interacts with nucleic acids and influences gene expression. Alternatively, it may be directed to the mitochondria, affecting cellular metabolism and energy production. Understanding the subcellular localization of this compound is essential for elucidating its role in cellular processes and its potential therapeutic applications.
Preparation Methods
Synthetic Routes and Reaction Conditions:
Direct Synthesis: tert-Butylphosphonic acid can be synthesized by the reaction of tert-butyl chloride with phosphorus trichloride, followed by hydrolysis. The reaction typically occurs in the presence of a base such as triethylamine.
Esterification: Another method involves the esterification of phosphonic acid with tert-butyl alcohol in the presence of a catalyst like sulfuric acid.
Industrial Production Methods: Industrial production of this compound often involves large-scale esterification and hydrolysis processes. The use of continuous flow reactors and optimized reaction conditions ensures high yield and purity of the product .
Chemical Reactions Analysis
Types of Reactions:
Oxidation: tert-Butylphosphonic acid can undergo oxidation reactions to form phosphonic acid derivatives with higher oxidation states.
Reduction: It can be reduced to form phosphine derivatives.
Substitution: The compound can participate in substitution reactions where the tert-butyl group is replaced by other functional groups.
Common Reagents and Conditions:
Oxidation: Common oxidizing agents include hydrogen peroxide and potassium permanganate.
Reduction: Reducing agents such as lithium aluminum hydride are used.
Substitution: Various halogenating agents and nucleophiles are employed in substitution reactions.
Major Products:
Oxidation: Phosphonic acid derivatives.
Reduction: Phosphine derivatives.
Substitution: Substituted phosphonic acids.
Scientific Research Applications
Chemistry:
Catalysis: tert-Butylphosphonic acid is used as a ligand in the preparation of catalysts for various organic reactions.
Material Science: It is employed in the synthesis of metal-organic frameworks and coordination polymers.
Biology and Medicine:
Drug Development: The compound is investigated for its potential use in drug delivery systems and as a building block for pharmaceuticals.
Industry:
Corrosion Inhibitors: It is used in formulations for corrosion inhibition in industrial processes.
Surface Treatment: The compound is applied in surface treatment processes to enhance the adhesion and durability of coatings.
Comparison with Similar Compounds
- Phenylphosphonic acid
- Methylphosphonic acid
- Octylphosphonic acid
Comparison:
- Phenylphosphonic acid: Unlike tert-butylphosphonic acid, phenylphosphonic acid has a phenyl group attached to the phosphorus atom, which affects its reactivity and applications.
- Methylphosphonic acid: This compound has a simpler structure with a methyl group, making it less bulky and more reactive in certain reactions.
- Octylphosphonic acid: The presence of a longer alkyl chain in octylphosphonic acid makes it more hydrophobic and suitable for applications in hydrophobic coatings .
Properties
IUPAC Name |
tert-butyl-dioxido-oxo-λ5-phosphane | |
---|---|---|
Details | Computed by LexiChem 2.6.6 (PubChem release 2019.06.18) | |
Source | PubChem | |
URL | https://pubchem.ncbi.nlm.nih.gov | |
Description | Data deposited in or computed by PubChem | |
InChI |
InChI=1S/C4H11O3P/c1-4(2,3)8(5,6)7/h1-3H3,(H2,5,6,7)/p-2 | |
Details | Computed by InChI 1.0.5 (PubChem release 2019.06.18) | |
Source | PubChem | |
URL | https://pubchem.ncbi.nlm.nih.gov | |
Description | Data deposited in or computed by PubChem | |
InChI Key |
OGDSVONAYZTTDA-UHFFFAOYSA-L | |
Details | Computed by InChI 1.0.5 (PubChem release 2019.06.18) | |
Source | PubChem | |
URL | https://pubchem.ncbi.nlm.nih.gov | |
Description | Data deposited in or computed by PubChem | |
Canonical SMILES |
CC(C)(C)P(=O)([O-])[O-] | |
Details | Computed by OEChem 2.1.5 (PubChem release 2019.06.18) | |
Source | PubChem | |
URL | https://pubchem.ncbi.nlm.nih.gov | |
Description | Data deposited in or computed by PubChem | |
Molecular Formula |
C4H9O3P-2 | |
Details | Computed by PubChem 2.1 (PubChem release 2019.06.18) | |
Source | PubChem | |
URL | https://pubchem.ncbi.nlm.nih.gov | |
Description | Data deposited in or computed by PubChem | |
DSSTOX Substance ID |
DTXSID101032342 | |
Record name | tert-Butylphosphonate | |
Source | EPA DSSTox | |
URL | https://comptox.epa.gov/dashboard/DTXSID101032342 | |
Description | DSSTox provides a high quality public chemistry resource for supporting improved predictive toxicology. | |
Molecular Weight |
136.09 g/mol | |
Details | Computed by PubChem 2.1 (PubChem release 2021.05.07) | |
Source | PubChem | |
URL | https://pubchem.ncbi.nlm.nih.gov | |
Description | Data deposited in or computed by PubChem | |
CAS No. |
4923-84-6, 99097-88-8 | |
Record name | NSC 222650 | |
Source | ChemIDplus | |
URL | https://pubchem.ncbi.nlm.nih.gov/substance/?source=chemidplus&sourceid=0004923846 | |
Description | ChemIDplus is a free, web search system that provides access to the structure and nomenclature authority files used for the identification of chemical substances cited in National Library of Medicine (NLM) databases, including the TOXNET system. | |
Record name | tert-Butylphosphonate | |
Source | EPA DSSTox | |
URL | https://comptox.epa.gov/dashboard/DTXSID101032342 | |
Description | DSSTox provides a high quality public chemistry resource for supporting improved predictive toxicology. | |
Retrosynthesis Analysis
AI-Powered Synthesis Planning: Our tool employs the Template_relevance Pistachio, Template_relevance Bkms_metabolic, Template_relevance Pistachio_ringbreaker, Template_relevance Reaxys, Template_relevance Reaxys_biocatalysis model, leveraging a vast database of chemical reactions to predict feasible synthetic routes.
One-Step Synthesis Focus: Specifically designed for one-step synthesis, it provides concise and direct routes for your target compounds, streamlining the synthesis process.
Accurate Predictions: Utilizing the extensive PISTACHIO, BKMS_METABOLIC, PISTACHIO_RINGBREAKER, REAXYS, REAXYS_BIOCATALYSIS database, our tool offers high-accuracy predictions, reflecting the latest in chemical research and data.
Strategy Settings
Precursor scoring | Relevance Heuristic |
---|---|
Min. plausibility | 0.01 |
Model | Template_relevance |
Template Set | Pistachio/Bkms_metabolic/Pistachio_ringbreaker/Reaxys/Reaxys_biocatalysis |
Top-N result to add to graph | 6 |
Feasible Synthetic Routes
Q1: What is the molecular formula and weight of tert-butylphosphonic acid?
A1: this compound, often denoted as tBuPO3H2, has the molecular formula C4H11O3P and a molecular weight of 138.10 g/mol.
Q2: What spectroscopic techniques are commonly employed to characterize this compound?
A2: Various spectroscopic methods are useful for characterizing this compound. These include: * Nuclear Magnetic Resonance (NMR) Spectroscopy: Both ¹H and ³¹P NMR are valuable for identifying and studying the compound in solution. ³¹P NMR is particularly useful for studying phosphorous-containing compounds like this compound [, , , ]. * Infrared (IR) Spectroscopy: IR spectroscopy provides information about the functional groups present in the molecule, such as P=O and P-OH vibrations [, ]. * Mass Spectrometry (MS): Techniques like electrospray ionization mass spectrometry (ESI-MS) can be used to determine the molecular weight and analyze fragment ions, providing insights into the compound's structure and fragmentation patterns [, ].
Q3: How does this compound interact with metal ions?
A3: this compound acts as a ligand, readily coordinating to various metal ions. The phosphonate group ([tBuPO3]2-) can bind in a variety of modes, including double or triple bridging or a combination of both. []. This versatility allows for the formation of diverse metal-organic frameworks with interesting structural and magnetic properties [, , , , , , , ].
Q4: What is the significance of this compound in materials science?
A4: this compound plays a crucial role in material science due to its ability to form robust self-assembled monolayers (SAMs) on metal oxide surfaces, particularly titanium dioxide (TiO2) [, ]. This property makes it valuable for surface modification, enhancing the compatibility of TiO2 with organic polymers and improving the performance of materials in applications such as capacitors [, ].
Q5: Can this compound be used to control the size of nanoparticles?
A5: Yes, studies have demonstrated that this compound can regulate the growth and size of ZnO nanoparticles during their synthesis. By adjusting the ratio of this compound to zinc acetate, researchers can achieve control over the final size of the ZnO nanocrystals [].
Q6: How does this compound self-associate in solution?
A6: this compound exhibits interesting self-association behavior in solution. In polar aprotic solvents, it has been shown to form highly symmetric cage-like tetramers. These tetramers are stabilized by eight O-H···O hydrogen bonds, creating a stable and well-defined structure [].
Q7: Can this compound be used to create larger molecular cages?
A7: Research has shown that this compound can be combined with boronic acids to synthesize borophosphonate cages. These cages have a heterocubane structure and exhibit dynamic behavior, undergoing exchange reactions at room temperature. This makes them promising building blocks for dynamic covalent chemistry [].
Q8: How does the choice of solvent influence the formation of metal phosphonate structures?
A8: Solvent choice significantly impacts the assembly and nuclearity of metal phosphonate structures. For instance, using methanol instead of acetonitrile in the reaction of vanadium trichloride with this compound resulted in the formation of a dinuclear vanadium(V) complex instead of dinuclear vanadium(IV) complexes [].
Q9: Does this compound exhibit any catalytic properties?
A9: Research suggests that certain titanium organophosphonate complexes incorporating this compound can function as effective and selective catalysts for olefin epoxidation reactions. These complexes have shown promising catalytic activity under both homogeneous and heterogeneous reaction conditions [].
Q10: How is computational chemistry employed to study this compound?
A10: Computational methods, particularly density functional theory (DFT), have proven to be invaluable tools in the study of this compound and its interactions. DFT calculations have been used to: * Study the self-association of this compound: These calculations provided insights into the stability and hydrogen bonding patterns of the cage-like tetramers formed in solution [, ]. * Investigate the interaction of this compound with surfaces: DFT simulations have been used to analyze the binding energies and preferred binding modes of this compound on the TiO2 surface of barium titanate, aiding in the understanding of surface functionalization []. * Calculate the interaction energies in molecular salts: Theoretical calculations can evaluate the stability of salts formed by the co-crystallization of this compound with other molecules, providing further support for experimental findings [].
Disclaimer and Information on In-Vitro Research Products
Please be aware that all articles and product information presented on BenchChem are intended solely for informational purposes. The products available for purchase on BenchChem are specifically designed for in-vitro studies, which are conducted outside of living organisms. In-vitro studies, derived from the Latin term "in glass," involve experiments performed in controlled laboratory settings using cells or tissues. It is important to note that these products are not categorized as medicines or drugs, and they have not received approval from the FDA for the prevention, treatment, or cure of any medical condition, ailment, or disease. We must emphasize that any form of bodily introduction of these products into humans or animals is strictly prohibited by law. It is essential to adhere to these guidelines to ensure compliance with legal and ethical standards in research and experimentation.