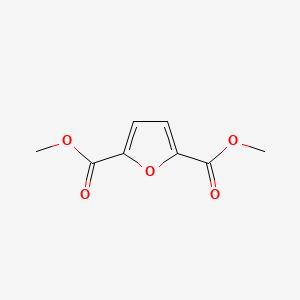
Dimethyl furan-2,5-dicarboxylate
Overview
Description
Dimethyl furan-2,5-dicarboxylate is an organic compound derived from furan, a heterocyclic aromatic compound. It is a diester of furan-2,5-dicarboxylic acid and is known for its potential applications in the production of bio-based polymers. This compound is gaining attention due to its role in creating sustainable and environmentally friendly materials.
Mechanism of Action
Dimethyl furan-2,5-dicarboxylate (DMFDC)
is a structural analog of diacids . It’s a bio-based monomer used in the synthesis of polyamides . Here’s an overview of its mechanism of action:
Target of Action
The primary target of DMFDC is the synthesis of polyamides . Polyamides are engineering thermoplastics employed in several demanding applications .
Mode of Action
DMFDC is prepared by esterification of furan-2,5-dicarboxylic acid (FDCA) with methanol in the presence of an acid (H2SO4) . It interacts with its targets through ester amidation reactions .
Biochemical Pathways
DMFDC is involved in the synthesis of a semi-aromatic polyamide, poly (hexamethylene furanamide) (PA6F), using melt polycondensation in the presence of two catalysts: Ti-isopropoxide (TIPT) and Ti-citrate (TIC) . The reaction results in PA6Fs with improved molecular weights and glass transition temperatures .
Pharmacokinetics
Its properties such as molecular weight and stability play a crucial role in its effectiveness in the synthesis process .
Result of Action
The resultant polymer shows high glass transition temperature (130 °C) and elastic modulus (3.5 GPa), and comparable thermal stability to its structural analogue poly (hexamethylene terephthalamide) (PA6T) .
Action Environment
The synthesis of DMFDC and its subsequent use in the creation of polyamides can be influenced by environmental factors such as temperature and the presence of catalysts . For instance, incorporating a slight excess (4.5 mol%) of hexamethylenediamine (HMDA) in the feed, results in further enhancement in the glass transition temperature .
Biochemical Analysis
Biochemical Properties
Dimethyl furan-2,5-dicarboxylate plays a crucial role in various biochemical reactions. It interacts with enzymes such as esterases and hydrolases, which catalyze the hydrolysis of ester bonds. These interactions are essential for the breakdown and utilization of this compound in metabolic pathways. Additionally, it can interact with proteins and other biomolecules, influencing their structure and function .
Cellular Effects
This compound has been shown to affect various types of cells and cellular processes. It can influence cell signaling pathways, gene expression, and cellular metabolism. For instance, it may modulate the activity of transcription factors, leading to changes in gene expression profiles. Furthermore, this compound can impact cellular metabolism by altering the flux of metabolites through different pathways .
Molecular Mechanism
The molecular mechanism of this compound involves its binding interactions with biomolecules. It can act as an enzyme inhibitor or activator, depending on the specific enzyme and context. For example, it may inhibit certain hydrolases, preventing the breakdown of ester bonds. Additionally, this compound can influence gene expression by interacting with transcription factors and other regulatory proteins .
Temporal Effects in Laboratory Settings
In laboratory settings, the effects of this compound can change over time. Its stability and degradation are important factors to consider. Studies have shown that this compound is relatively stable under standard laboratory conditions, but it can degrade over extended periods. Long-term exposure to this compound may lead to changes in cellular function, including alterations in gene expression and metabolic activity .
Dosage Effects in Animal Models
The effects of this compound vary with different dosages in animal models. At low doses, it may have minimal impact on cellular function, while higher doses can lead to significant changes. Threshold effects have been observed, where a certain dosage is required to elicit a measurable response. At high doses, this compound may exhibit toxic or adverse effects, including cellular damage and disruption of metabolic processes .
Metabolic Pathways
This compound is involved in several metabolic pathways. It interacts with enzymes such as esterases and hydrolases, which catalyze its breakdown into smaller metabolites. These metabolites can then enter various metabolic pathways, influencing metabolic flux and metabolite levels. The compound’s involvement in these pathways highlights its importance in cellular metabolism .
Transport and Distribution
Within cells and tissues, this compound is transported and distributed through specific mechanisms. It can interact with transporters and binding proteins, facilitating its movement across cellular membranes. These interactions can affect its localization and accumulation within different cellular compartments. Understanding the transport and distribution of this compound is crucial for elucidating its cellular effects .
Subcellular Localization
This compound exhibits specific subcellular localization, which can influence its activity and function. It may be directed to particular compartments or organelles through targeting signals or post-translational modifications. For example, it could localize to the endoplasmic reticulum or mitochondria, where it can interact with specific enzymes and proteins. The subcellular localization of this compound is essential for understanding its role in cellular processes .
Preparation Methods
Synthetic Routes and Reaction Conditions: Dimethyl furan-2,5-dicarboxylate is typically synthesized through the esterification of furan-2,5-dicarboxylic acid with methanol. This reaction is often catalyzed by acids such as sulfuric acid or p-toluenesulfonic acid. The reaction conditions usually involve heating the reactants under reflux to facilitate the esterification process .
Industrial Production Methods: In industrial settings, the production of this compound can be scaled up by using continuous flow reactors. This method allows for better control over reaction conditions and improves the yield and purity of the product. The crude product is often purified through crystallization and vacuum distillation .
Chemical Reactions Analysis
Types of Reactions: Dimethyl furan-2,5-dicarboxylate undergoes various chemical reactions, including:
Oxidation: It can be oxidized to form furan-2,5-dicarboxylic acid.
Reduction: Hydrogenation of this compound can produce dimethyl tetrahydrofuran-2,5-dicarboxylate.
Substitution: It can participate in substitution reactions where the ester groups are replaced by other functional groups.
Common Reagents and Conditions:
Oxidation: Common oxidizing agents include potassium permanganate and hydrogen peroxide.
Substitution: Reagents like alkyl halides or acyl chlorides can be used under basic or acidic conditions.
Major Products:
Oxidation: Furan-2,5-dicarboxylic acid.
Reduction: Dimethyl tetrahydrofuran-2,5-dicarboxylate.
Substitution: Various substituted furan derivatives depending on the reagents used.
Scientific Research Applications
Dimethyl furan-2,5-dicarboxylate has several applications in scientific research:
Biology: Its derivatives are studied for their potential biological activities, including antimicrobial and anticancer properties.
Medicine: Research is ongoing to explore its use in drug delivery systems due to its biocompatibility and biodegradability.
Comparison with Similar Compounds
Dimethyl furan-2,5-dicarboxylate can be compared with other similar compounds such as:
Dimethyl terephthalate: Both are diesters used in polymer production, but this compound is derived from renewable resources, making it more sustainable.
Dimethyl isophthalate: Similar in structure but differs in the position of the ester groups, affecting the properties of the resulting polymers.
Dimethyl succinate: Another diester used in polymer synthesis, but with a different backbone structure, leading to different polymer properties.
Uniqueness: this compound stands out due to its bio-based origin and its potential to replace petroleum-derived monomers in the production of sustainable polymers .
Properties
IUPAC Name |
dimethyl furan-2,5-dicarboxylate | |
---|---|---|
Source | PubChem | |
URL | https://pubchem.ncbi.nlm.nih.gov | |
Description | Data deposited in or computed by PubChem | |
InChI |
InChI=1S/C8H8O5/c1-11-7(9)5-3-4-6(13-5)8(10)12-2/h3-4H,1-2H3 | |
Source | PubChem | |
URL | https://pubchem.ncbi.nlm.nih.gov | |
Description | Data deposited in or computed by PubChem | |
InChI Key |
UWQOPFRNDNVUOA-UHFFFAOYSA-N | |
Source | PubChem | |
URL | https://pubchem.ncbi.nlm.nih.gov | |
Description | Data deposited in or computed by PubChem | |
Canonical SMILES |
COC(=O)C1=CC=C(O1)C(=O)OC | |
Source | PubChem | |
URL | https://pubchem.ncbi.nlm.nih.gov | |
Description | Data deposited in or computed by PubChem | |
Molecular Formula |
C8H8O5 | |
Source | PubChem | |
URL | https://pubchem.ncbi.nlm.nih.gov | |
Description | Data deposited in or computed by PubChem | |
DSSTOX Substance ID |
DTXSID50307531 | |
Record name | dimethyl furan-2,5-dicarboxylate | |
Source | EPA DSSTox | |
URL | https://comptox.epa.gov/dashboard/DTXSID50307531 | |
Description | DSSTox provides a high quality public chemistry resource for supporting improved predictive toxicology. | |
Molecular Weight |
184.15 g/mol | |
Source | PubChem | |
URL | https://pubchem.ncbi.nlm.nih.gov | |
Description | Data deposited in or computed by PubChem | |
CAS No. |
4282-32-0 | |
Record name | 2,5-Dimethyl 2,5-furandicarboxylate | |
Source | CAS Common Chemistry | |
URL | https://commonchemistry.cas.org/detail?cas_rn=4282-32-0 | |
Description | CAS Common Chemistry is an open community resource for accessing chemical information. Nearly 500,000 chemical substances from CAS REGISTRY cover areas of community interest, including common and frequently regulated chemicals, and those relevant to high school and undergraduate chemistry classes. This chemical information, curated by our expert scientists, is provided in alignment with our mission as a division of the American Chemical Society. | |
Explanation | The data from CAS Common Chemistry is provided under a CC-BY-NC 4.0 license, unless otherwise stated. | |
Record name | NSC 191940 | |
Source | ChemIDplus | |
URL | https://pubchem.ncbi.nlm.nih.gov/substance/?source=chemidplus&sourceid=0004282320 | |
Description | ChemIDplus is a free, web search system that provides access to the structure and nomenclature authority files used for the identification of chemical substances cited in National Library of Medicine (NLM) databases, including the TOXNET system. | |
Record name | 4282-32-0 | |
Source | DTP/NCI | |
URL | https://dtp.cancer.gov/dtpstandard/servlet/dwindex?searchtype=NSC&outputformat=html&searchlist=191940 | |
Description | The NCI Development Therapeutics Program (DTP) provides services and resources to the academic and private-sector research communities worldwide to facilitate the discovery and development of new cancer therapeutic agents. | |
Explanation | Unless otherwise indicated, all text within NCI products is free of copyright and may be reused without our permission. Credit the National Cancer Institute as the source. | |
Record name | dimethyl furan-2,5-dicarboxylate | |
Source | EPA DSSTox | |
URL | https://comptox.epa.gov/dashboard/DTXSID50307531 | |
Description | DSSTox provides a high quality public chemistry resource for supporting improved predictive toxicology. | |
Record name | dimethyl furan-2,5-dicarboxylate | |
Source | European Chemicals Agency (ECHA) | |
URL | https://echa.europa.eu/information-on-chemicals | |
Description | The European Chemicals Agency (ECHA) is an agency of the European Union which is the driving force among regulatory authorities in implementing the EU's groundbreaking chemicals legislation for the benefit of human health and the environment as well as for innovation and competitiveness. | |
Explanation | Use of the information, documents and data from the ECHA website is subject to the terms and conditions of this Legal Notice, and subject to other binding limitations provided for under applicable law, the information, documents and data made available on the ECHA website may be reproduced, distributed and/or used, totally or in part, for non-commercial purposes provided that ECHA is acknowledged as the source: "Source: European Chemicals Agency, http://echa.europa.eu/". Such acknowledgement must be included in each copy of the material. ECHA permits and encourages organisations and individuals to create links to the ECHA website under the following cumulative conditions: Links can only be made to webpages that provide a link to the Legal Notice page. | |
Retrosynthesis Analysis
AI-Powered Synthesis Planning: Our tool employs the Template_relevance Pistachio, Template_relevance Bkms_metabolic, Template_relevance Pistachio_ringbreaker, Template_relevance Reaxys, Template_relevance Reaxys_biocatalysis model, leveraging a vast database of chemical reactions to predict feasible synthetic routes.
One-Step Synthesis Focus: Specifically designed for one-step synthesis, it provides concise and direct routes for your target compounds, streamlining the synthesis process.
Accurate Predictions: Utilizing the extensive PISTACHIO, BKMS_METABOLIC, PISTACHIO_RINGBREAKER, REAXYS, REAXYS_BIOCATALYSIS database, our tool offers high-accuracy predictions, reflecting the latest in chemical research and data.
Strategy Settings
Precursor scoring | Relevance Heuristic |
---|---|
Min. plausibility | 0.01 |
Model | Template_relevance |
Template Set | Pistachio/Bkms_metabolic/Pistachio_ringbreaker/Reaxys/Reaxys_biocatalysis |
Top-N result to add to graph | 6 |
Feasible Synthetic Routes
Disclaimer and Information on In-Vitro Research Products
Please be aware that all articles and product information presented on BenchChem are intended solely for informational purposes. The products available for purchase on BenchChem are specifically designed for in-vitro studies, which are conducted outside of living organisms. In-vitro studies, derived from the Latin term "in glass," involve experiments performed in controlled laboratory settings using cells or tissues. It is important to note that these products are not categorized as medicines or drugs, and they have not received approval from the FDA for the prevention, treatment, or cure of any medical condition, ailment, or disease. We must emphasize that any form of bodily introduction of these products into humans or animals is strictly prohibited by law. It is essential to adhere to these guidelines to ensure compliance with legal and ethical standards in research and experimentation.