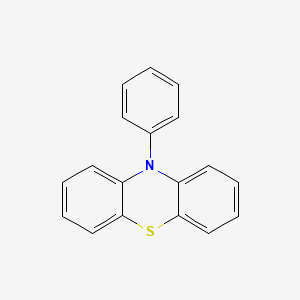
10-Phenyl-10h-phenothiazine
Overview
Description
10-Phenyl-10H-phenothiazine is a heterocyclic compound featuring a phenothiazine core substituted with a phenyl group at the nitrogen atom. Its structure combines a sulfur- and nitrogen-containing tricyclic system with an aromatic phenyl substituent, enabling unique electronic and photophysical properties. This compound has garnered significant attention in materials science due to its applications in organic light-emitting diodes (OLEDs), room-temperature phosphorescence (RTP), and dye-sensitized solar cells (DSSCs). The phenyl group enhances steric hindrance and electronic delocalization, making it a versatile scaffold for tuning optoelectronic behavior. Its derivatives are widely explored for their aggregation-induced emission (AIE) and charge-transfer characteristics.
Preparation Methods
Synthetic Routes and Reaction Conditions: 10-Phenyl-10H-phenothiazine can be synthesized through several methods. One common approach involves the condensation of phenothiazine with phenyl halides under basic conditions. Another method includes the use of palladium-catalyzed cross-coupling reactions, such as the Suzuki-Miyaura coupling, where phenothiazine is reacted with phenylboronic acid in the presence of a palladium catalyst .
Industrial Production Methods: In industrial settings, the synthesis of this compound often involves large-scale palladium-catalyzed cross-coupling reactions due to their efficiency and high yield. The reaction is typically carried out in a solvent such as tetrahydrofuran (THF) or dimethylformamide (DMF) at elevated temperatures .
Chemical Reactions Analysis
Types of Reactions: 10-Phenyl-10H-phenothiazine undergoes various chemical reactions, including:
Oxidation: The compound can be oxidized to form phenothiazine sulfoxides and sulfones.
Reduction: Reduction reactions can convert the compound to its corresponding dihydro derivatives.
Substitution: Electrophilic substitution reactions can introduce various substituents onto the phenothiazine core.
Common Reagents and Conditions:
Oxidation: Common oxidizing agents include hydrogen peroxide and m-chloroperbenzoic acid.
Reduction: Reducing agents such as lithium aluminum hydride or sodium borohydride are typically used.
Substitution: Electrophilic reagents like halogens or nitro compounds are used under acidic or basic conditions.
Major Products:
Oxidation: Phenothiazine sulfoxides and sulfones.
Reduction: Dihydro derivatives of this compound.
Substitution: Various substituted phenothiazine derivatives.
Scientific Research Applications
10-Phenyl-10H-phenothiazine has a wide range of applications in scientific research:
Mechanism of Action
The mechanism of action of 10-Phenyl-10H-phenothiazine involves its ability to act as a photocatalyst. Upon absorption of light, the compound undergoes electronic excitation, leading to the formation of reactive intermediates that can participate in various chemical reactions. The molecular targets and pathways involved include the generation of singlet oxygen and other reactive oxygen species, which can facilitate oxidation reactions .
Comparison with Similar Compounds
Structural Modifications and Electronic Properties
The photophysical and electronic properties of 10-phenyl-10H-phenothiazine derivatives are highly sensitive to substituent effects. Key comparisons include:
Key Findings :
- Substituent Position : Methyl groups at axial positions (e.g., O-PTZ-1Me-H) enhance RTP by restricting molecular motion, while equatorial substitutions (e.g., O-PTZ-H-2Me) reduce efficiency.
- Electron-Donating Groups : Methoxy substituents (4a, 4b) increase solubility and alter melting points but marginally affect sulfur content.
- Exciplex Systems : BPC@PPZ demonstrates superior quantum yield (36.3%) due to charge-transfer interactions.
- Solar Cell Applications : JW1 achieves 7.2% efficiency in DSSCs via enhanced electron delocalization.
Thermal and Stability Comparisons
- Melting Points : Derivatives with bulky substituents (e.g., 4a: 160°C vs. 4b: 209°C) exhibit higher thermal stability due to increased molecular rigidity.
- Photostability: Phenothiazine-5,5-dioxide derivatives (e.g., O-PTZ-H) show improved stability under UV irradiation compared to non-oxidized analogs.
Optoelectronic Performance
- OLEDs: 10-Phenylphenothiazine derivatives with sulfonyl groups (e.g., 5,5-dioxide) exhibit aggregation-induced delayed fluorescence (AIDF) with Commission Internationale de l’Éclairage (CIE) coordinates of (0.16, 0.07), outperforming carbazole-based emitters.
- RTP Lifetimes : Methyl-substituted derivatives (e.g., O-PTZ-2Me-H) achieve microsecond-scale RTP lifetimes, while halogenated analogs (CS-Br, CS-Cl) show reduced performance due to heavy atom effects.
Biological Activity
10-Phenyl-10H-phenothiazine (PTH) is a compound belonging to the phenothiazine family, which has garnered attention due to its diverse biological activities and potential therapeutic applications. This article explores the biological activity of PTH, focusing on its pharmacological properties, mechanisms of action, and implications for drug development.
Overview of this compound
PTH is characterized by a phenothiazine backbone with a phenyl substituent at the 10 position. This structural modification enhances its photocatalytic properties while retaining significant biological activity similar to other derivatives in the phenothiazine class. The compound exhibits interactions with neurotransmitter systems, particularly dopamine receptors, contributing to its antipsychotic effects.
Pharmacological Properties
The biological activity of PTH is multifaceted, with research indicating its potential in various therapeutic areas:
- Antipsychotic Activity : PTH interacts with dopamine D₂ receptors, similar to other phenothiazines. This interaction has been linked to its antipsychotic properties, making it a candidate for treating psychiatric disorders .
- Antimicrobial Effects : Studies have shown that PTH and its derivatives possess antimicrobial properties against various pathogens. This suggests potential applications in developing new antimicrobial therapies .
- Antitumor Activity : PTH has demonstrated significant antitumor effects in vitro. For instance, phenothiazine-ketone hybrids derived from PTH exhibited growth inhibition in human tumor cell lines at low nanomolar concentrations (GI50: 1.8–6.5 nM) .
The mechanisms through which PTH exerts its biological effects include:
- Receptor Binding : The binding affinity of PTH to dopamine receptors plays a crucial role in its antipsychotic effects. Research indicates that structural modifications can influence this binding affinity and, consequently, the compound's efficacy .
- Electron Transfer Processes : As a photocatalyst, PTH can undergo electron transfer processes upon light absorption, facilitating various chemical transformations. This property is leveraged in synthetic organic chemistry for reactions that are otherwise challenging to achieve without suitable catalysts .
Case Studies
Several studies have investigated the biological activity of PTH and its derivatives:
- Antitumor Efficacy : A study screened phenothiazine-ketone derivatives for their ability to inhibit tubulin polymerization and farnesyltransferase (FTase), critical targets in cancer therapy. Among these compounds, specific derivatives showed potent inhibition against cancer cell proliferation .
- Antimicrobial Assessment : Research evaluated the antimicrobial activity of new substituted 10H-phenothiazines against various bacterial strains. The results indicated that minor modifications in the substitution pattern significantly influenced biological activity, highlighting the importance of structural optimization .
Comparative Analysis of Phenothiazine Derivatives
The following table summarizes key properties and activities of various phenothiazine derivatives compared to this compound:
Compound Name | Structure Type | Key Properties |
---|---|---|
This compound | Parent Compound | Antipsychotic effects; photocatalytic properties |
Chlorpromazine | Phenothiazine Derivative | Antipsychotic; high D₂ receptor affinity |
Promethazine | Phenothiazine Derivative | Antihistamine; used for allergies |
Methylene Blue | Phenothiazine Derivative | Antimalarial; used as a dye |
Thionine | Phenothiazine Derivative | Staining agent; used in histology |
Q & A
Basic Research Questions
Q. What are the optimal synthetic routes for preparing 10-phenyl-10H-phenothiazine derivatives, and how do reaction conditions influence yield?
- Methodology : The synthesis of phenothiazine derivatives typically involves nucleophilic substitution or coupling reactions. For example, methyl 4-[2-(10H-phenothiazin-10-yl)ethyl]benzoate (20) is synthesized via a TFA/Et3SiH-mediated reaction under nitrogen at 0°C, followed by NaOH neutralization and column chromatography . Yield optimization can be explored by varying catalysts (e.g., Pd-based catalysts for cross-coupling), solvents (polar vs. nonpolar), and temperature. Monitoring reaction progress via TLC or HPLC is critical.
Q. Which spectroscopic and crystallographic techniques are most effective for characterizing this compound derivatives?
- Methodology :
- NMR : ¹H/¹³C NMR identifies substituent positions and confirms purity (e.g., ¹³C signals at 143.6 ppm for C-10a’’’ in HDAC inhibitors ).
- XRD : Single-crystal X-ray diffraction (e.g., triclinic system, space group P1 for 10-hexyl derivatives) provides bond lengths, angles, and torsion angles (e.g., C–C bonds: 1.463–1.524 Å) .
- MS : High-resolution mass spectrometry validates molecular formulas (e.g., m/z 384.0568 for [(C19H15ClN3O2S)+] ).
Q. How can solubility challenges in phenothiazine derivatives be addressed during purification?
- Methodology : Poor solubility in nonpolar solvents (e.g., petroleum ether) necessitates gradient elution in column chromatography using mixtures like hexane/ethyl acetate . Recrystallization from ethanol or acetic acid is effective for crystalline derivatives .
Q. What are common impurities in phenothiazine synthesis, and how are they identified?
- Methodology : Byproducts like unreacted starting materials or oxidation products (e.g., sulfoxides) are detected via HPLC-MS. Impurity profiling in related compounds (e.g., chlorpromazine derivatives) involves comparing retention times and spectral data .
Q. How does the electronic structure of this compound influence its reactivity?
- Methodology : The electron-rich sulfur and nitrogen atoms enable electrophilic substitution at the para positions. DFT calculations can map electron density distributions, while cyclic voltammetry assesses redox behavior (e.g., oxidation potentials relevant to charge-transfer applications) .
Advanced Research Questions
Q. How can factorial design optimize reaction parameters for synthesizing this compound-based HDAC inhibitors?
- Methodology : A 2³ factorial design evaluates variables like temperature (25–60°C), catalyst loading (5–15 mol%), and solvent polarity (DMF vs. THF). Response surface methodology (RSM) identifies interactions affecting yield and selectivity. For example, higher temperatures may accelerate coupling but increase side reactions .
Q. What strategies improve selectivity of phenothiazine-based HDAC inhibitors against isoforms like HDAC6 vs. HDAC1?
- Methodology :
- Structural Modification : Introduce bulkier substituents (e.g., 4-nitrophenyl ethynyl groups) to exploit HDAC6’s larger active site .
- Bioactivity Assays : Use isoform-specific fluorogenic substrates (e.g., tubulin deacetylation for HDAC6) and compare IC50 values .
Q. How do discrepancies between experimental (XRD) and computational (DFT) bond angles arise in phenothiazine derivatives?
- Methodology : XRD provides empirical geometries (e.g., C–S–C angles: 98.781° in 10-hexyl derivatives ), while DFT approximations may neglect crystal packing effects. Hybrid functional simulations (e.g., B3LYP-D3) with dispersion corrections reduce such gaps .
Q. Can machine learning predict crystallization outcomes for this compound cocrystals?
- Methodology : Train ML models on datasets of known cocrystals (e.g., API-coformer interactions) using descriptors like hydrogen-bond propensity and molecular symmetry. Validate predictions via PXRD and DSC (e.g., cocrystal with indenyl derivatives ).
Q. How do torsional strain and non-covalent interactions affect the photophysical properties of phenothiazine derivatives?
Properties
IUPAC Name |
10-phenylphenothiazine | |
---|---|---|
Source | PubChem | |
URL | https://pubchem.ncbi.nlm.nih.gov | |
Description | Data deposited in or computed by PubChem | |
InChI |
InChI=1S/C18H13NS/c1-2-8-14(9-3-1)19-15-10-4-6-12-17(15)20-18-13-7-5-11-16(18)19/h1-13H | |
Source | PubChem | |
URL | https://pubchem.ncbi.nlm.nih.gov | |
Description | Data deposited in or computed by PubChem | |
InChI Key |
WSEFYHOJDVVORU-UHFFFAOYSA-N | |
Source | PubChem | |
URL | https://pubchem.ncbi.nlm.nih.gov | |
Description | Data deposited in or computed by PubChem | |
Canonical SMILES |
C1=CC=C(C=C1)N2C3=CC=CC=C3SC4=CC=CC=C42 | |
Source | PubChem | |
URL | https://pubchem.ncbi.nlm.nih.gov | |
Description | Data deposited in or computed by PubChem | |
Molecular Formula |
C18H13NS | |
Source | PubChem | |
URL | https://pubchem.ncbi.nlm.nih.gov | |
Description | Data deposited in or computed by PubChem | |
DSSTOX Substance ID |
DTXSID70221760 | |
Record name | Phenothiazine, 10-phenyl- | |
Source | EPA DSSTox | |
URL | https://comptox.epa.gov/dashboard/DTXSID70221760 | |
Description | DSSTox provides a high quality public chemistry resource for supporting improved predictive toxicology. | |
Molecular Weight |
275.4 g/mol | |
Source | PubChem | |
URL | https://pubchem.ncbi.nlm.nih.gov | |
Description | Data deposited in or computed by PubChem | |
CAS No. |
7152-42-3 | |
Record name | 10-Phenylphenothiazine | |
Source | CAS Common Chemistry | |
URL | https://commonchemistry.cas.org/detail?cas_rn=7152-42-3 | |
Description | CAS Common Chemistry is an open community resource for accessing chemical information. Nearly 500,000 chemical substances from CAS REGISTRY cover areas of community interest, including common and frequently regulated chemicals, and those relevant to high school and undergraduate chemistry classes. This chemical information, curated by our expert scientists, is provided in alignment with our mission as a division of the American Chemical Society. | |
Explanation | The data from CAS Common Chemistry is provided under a CC-BY-NC 4.0 license, unless otherwise stated. | |
Record name | N-Phenylphenothiazine | |
Source | ChemIDplus | |
URL | https://pubchem.ncbi.nlm.nih.gov/substance/?source=chemidplus&sourceid=0007152423 | |
Description | ChemIDplus is a free, web search system that provides access to the structure and nomenclature authority files used for the identification of chemical substances cited in National Library of Medicine (NLM) databases, including the TOXNET system. | |
Record name | 10-Phenylphenothiazine | |
Source | DTP/NCI | |
URL | https://dtp.cancer.gov/dtpstandard/servlet/dwindex?searchtype=NSC&outputformat=html&searchlist=23181 | |
Description | The NCI Development Therapeutics Program (DTP) provides services and resources to the academic and private-sector research communities worldwide to facilitate the discovery and development of new cancer therapeutic agents. | |
Explanation | Unless otherwise indicated, all text within NCI products is free of copyright and may be reused without our permission. Credit the National Cancer Institute as the source. | |
Record name | Phenothiazine, 10-phenyl- | |
Source | EPA DSSTox | |
URL | https://comptox.epa.gov/dashboard/DTXSID70221760 | |
Description | DSSTox provides a high quality public chemistry resource for supporting improved predictive toxicology. | |
Record name | N-PHENYLPHENOTHIAZINE | |
Source | FDA Global Substance Registration System (GSRS) | |
URL | https://gsrs.ncats.nih.gov/ginas/app/beta/substances/BII1EYP5EW | |
Description | The FDA Global Substance Registration System (GSRS) enables the efficient and accurate exchange of information on what substances are in regulated products. Instead of relying on names, which vary across regulatory domains, countries, and regions, the GSRS knowledge base makes it possible for substances to be defined by standardized, scientific descriptions. | |
Explanation | Unless otherwise noted, the contents of the FDA website (www.fda.gov), both text and graphics, are not copyrighted. They are in the public domain and may be republished, reprinted and otherwise used freely by anyone without the need to obtain permission from FDA. Credit to the U.S. Food and Drug Administration as the source is appreciated but not required. | |
Synthesis routes and methods
Procedure details
Retrosynthesis Analysis
AI-Powered Synthesis Planning: Our tool employs the Template_relevance Pistachio, Template_relevance Bkms_metabolic, Template_relevance Pistachio_ringbreaker, Template_relevance Reaxys, Template_relevance Reaxys_biocatalysis model, leveraging a vast database of chemical reactions to predict feasible synthetic routes.
One-Step Synthesis Focus: Specifically designed for one-step synthesis, it provides concise and direct routes for your target compounds, streamlining the synthesis process.
Accurate Predictions: Utilizing the extensive PISTACHIO, BKMS_METABOLIC, PISTACHIO_RINGBREAKER, REAXYS, REAXYS_BIOCATALYSIS database, our tool offers high-accuracy predictions, reflecting the latest in chemical research and data.
Strategy Settings
Precursor scoring | Relevance Heuristic |
---|---|
Min. plausibility | 0.01 |
Model | Template_relevance |
Template Set | Pistachio/Bkms_metabolic/Pistachio_ringbreaker/Reaxys/Reaxys_biocatalysis |
Top-N result to add to graph | 6 |
Feasible Synthetic Routes
Disclaimer and Information on In-Vitro Research Products
Please be aware that all articles and product information presented on BenchChem are intended solely for informational purposes. The products available for purchase on BenchChem are specifically designed for in-vitro studies, which are conducted outside of living organisms. In-vitro studies, derived from the Latin term "in glass," involve experiments performed in controlled laboratory settings using cells or tissues. It is important to note that these products are not categorized as medicines or drugs, and they have not received approval from the FDA for the prevention, treatment, or cure of any medical condition, ailment, or disease. We must emphasize that any form of bodily introduction of these products into humans or animals is strictly prohibited by law. It is essential to adhere to these guidelines to ensure compliance with legal and ethical standards in research and experimentation.