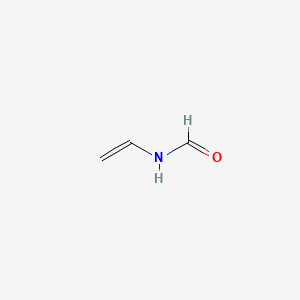
N-Vinylformamide
Overview
Description
N-Vinylformamide is a vinyl monomer with high reactivity and water solubility. It is known for its versatility in various applications, including the synthesis of specialty polymers with stimuli-responsive behavior. This compound is biocompatible and suitable for use in drug delivery systems, tissue engineering, and controlled-release applications .
Mechanism of Action
Target of Action
N-Vinylformamide (NVF) is a vinyl monomer with high reactivity and water solubility . It is primarily used as a monomer to synthesize specialty polymers with stimuli-responsive behavior . The primary targets of NVF are the polymer chains where it is incorporated during the polymerization process .
Mode of Action
NVF interacts with its targets through the process of free radical chain polymerization . In this process, NVF is grafted onto a base material, such as a bacterial cellulose sheet or other polymer chains . This grafting process enhances the properties of the base material, such as its adsorption affinity .
Biochemical Pathways
The biochemical pathways affected by NVF primarily involve the synthesis of poly(this compound) (PNVF) based hydrogels . These hydrogels are synthesized through free radical chain polymerization using NVF as a monomer . The resulting hydrogels exhibit stimuli-responsive behavior, which can be utilized in various applications .
Pharmacokinetics
The properties of nvf, such as its high reactivity and water solubility, suggest that it would have good bioavailability if used in a biological context .
Result of Action
The result of NVF’s action is the formation of specialty polymers with enhanced properties . For example, NVF can enhance the adsorption affinity of a membrane by inducing multiple hydrophilic and hydrogen bonding sites on the surface . Additionally, NVF can be used to synthesize PNVF-based hydrogels that can be used for controlled drug delivery .
Action Environment
The action of NVF is influenced by environmental factors such as temperature and pH . For instance, the rate of polymerization can be affected by temperature . Additionally, the properties of the resulting polymers, such as their swelling behavior and mechanical strength, can be influenced by the pH of the environment .
Biochemical Analysis
Biochemical Properties
N-Vinylformamide plays a significant role in biochemical reactions due to its ability to form polymers that interact with various biomolecules. It can interact with enzymes, proteins, and other biomolecules through hydrogen bonding and hydrophilic interactions. For example, this compound can be used to synthesize poly(this compound) hydrogels, which can interact with enzymes like lysozyme and proteins such as bovine serum albumin, enhancing their stability and activity .
Cellular Effects
This compound influences various types of cells and cellular processes. It has been shown to affect cell signaling pathways, gene expression, and cellular metabolism. For instance, this compound-based hydrogels can promote cell adhesion and proliferation, making them useful in tissue engineering applications. Additionally, these hydrogels can modulate the expression of genes involved in cell cycle regulation and apoptosis .
Molecular Mechanism
The molecular mechanism of this compound involves its ability to form hydrogen bonds and hydrophilic interactions with biomolecules. This compound can bind to enzymes and proteins, leading to enzyme inhibition or activation. For example, this compound can inhibit the activity of certain proteases by binding to their active sites, thereby preventing substrate binding and catalysis. Additionally, this compound can influence gene expression by interacting with transcription factors and other regulatory proteins .
Temporal Effects in Laboratory Settings
In laboratory settings, the effects of this compound can change over time due to its stability and degradation. This compound is relatively stable under physiological conditions, but it can undergo hydrolysis to form N-vinylamine, which can further interact with biomolecules. Long-term studies have shown that this compound-based hydrogels maintain their structural integrity and functionality over extended periods, making them suitable for sustained drug delivery and tissue engineering applications .
Dosage Effects in Animal Models
The effects of this compound vary with different dosages in animal models. At low doses, this compound-based hydrogels can promote tissue regeneration and wound healing without causing significant toxicity. At high doses, this compound can exhibit toxic effects, such as inducing oxidative stress and inflammation. Threshold effects have been observed, where the beneficial effects of this compound are maximized at optimal dosages, while adverse effects are minimized .
Metabolic Pathways
This compound is involved in various metabolic pathways, including its hydrolysis to form N-vinylamine. This compound can interact with enzymes such as hydrolases and oxidases, affecting metabolic flux and metabolite levels. For example, this compound can be metabolized by liver enzymes, leading to the formation of reactive intermediates that can further interact with cellular components .
Transport and Distribution
This compound is transported and distributed within cells and tissues through passive diffusion and active transport mechanisms. It can interact with transporters and binding proteins, influencing its localization and accumulation. For instance, this compound can be taken up by cells through endocytosis and distributed to various cellular compartments, including the cytoplasm and nucleus .
Subcellular Localization
The subcellular localization of this compound is influenced by its chemical properties and interactions with biomolecules. It can be directed to specific compartments or organelles through targeting signals and post-translational modifications. For example, this compound can accumulate in the endoplasmic reticulum and Golgi apparatus, where it can interact with enzymes involved in protein synthesis and modification .
Preparation Methods
N-Vinylformamide can be synthesized through several methods. One common method involves the reaction of hydroxyethyl formamide with a reactant containing at least one cyclic anhydride group to form an ester. This ester is then dissociated via heat in a thin film evaporation process to produce this compound and a compound containing at least one diacid group . Another method includes the copolymerization of this compound with acrylonitrile in aqueous solution, followed by amidinization of the copolymer . Additionally, reversible addition–fragmentation chain transfer (RAFT) polymerization of this compound has been reported, which involves reactions conducted in dimethyl sulfoxide at 35°C using a xanthate RAFT agent .
Chemical Reactions Analysis
N-Vinylformamide undergoes various chemical reactions, including polymerization and hydrolysis. The RAFT polymerization of this compound results in the formation of poly(this compound) with predetermined molar masses and low dispersities . The copolymerization of this compound with acrylonitrile and subsequent amidinization produces polyamidine, which exhibits high charge density and flocculation performance . Common reagents used in these reactions include xanthate RAFT agents and acrylonitrile. Major products formed from these reactions include poly(this compound) and polyamidine.
Scientific Research Applications
N-Vinylformamide has a wide range of scientific research applications. It is used as a monomer to synthesize specialty polymers with stimuli-responsive behavior, making it suitable for drug delivery systems, tissue engineering, and controlled-release applications . In the field of batteries, this compound is used to synthesize an aqueous binder for lithium titanate anodes, enhancing the stability and specific capacity of the electrodes . It is also employed in the fabrication of biocompatible coatings for medical devices and polypropylene membranes for continuous removal of organic micropollutants from water . Additionally, this compound-based hydrogels have been investigated for their potential in controlled drug delivery by altering the polymer composition to govern the amount and ratio of free to bound water contained in the hydrogels .
Comparison with Similar Compounds
N-Vinylformamide can be compared with other similar compounds such as N-Vinylcaprolactam, N,N-Dimethylformamide, and N-Isopropylacrylamide . N-Vinylcaprolactam is another vinyl monomer used in the synthesis of specialty polymers, while N,N-Dimethylformamide is a common solvent used in various chemical reactions. N-Isopropylacrylamide is known for its use in the synthesis of temperature-responsive hydrogels. This compound stands out due to its high reactivity, water solubility, and biocompatibility, making it suitable for a wide range of applications in drug delivery, tissue engineering, and water treatment.
Biological Activity
N-Vinylformamide (NVF) is a multifunctional monomer that has garnered attention for its biological activity, particularly in biomedical applications. This article explores the biological properties of NVF, focusing on its antimicrobial activity, biocompatibility, and potential therapeutic uses, supported by relevant research findings and case studies.
Overview of this compound
This compound is a vinyl monomer that can undergo polymerization to form poly(this compound) (PNVF). This polymer can be further hydrolyzed to produce poly(vinyl amine) (PVAm), which exhibits significant biological activity. The conversion of NVF to PNVF and subsequently to PVAm can be achieved through various hydrolysis methods, influencing the final properties of the polymers.
Antimicrobial Activity
Research Findings:
- Hydrolysis Effects : The hydrolysis of PNVF into PVAm significantly enhances its antimicrobial properties. Studies have shown that PVAm derived from acidic hydrolysis exhibits potent antimicrobial activity against various pathogens, including Escherichia coli, Staphylococcus aureus, and Candida albicans. The antimicrobial efficacy was reported to be 10-20 times greater than that of many conventional antimicrobial agents .
- Mechanism of Action : The primary amine groups formed during the hydrolysis process are believed to interact with microbial cell membranes, leading to cell lysis and death. This mechanism highlights the potential of PVAm in developing antimicrobial coatings and materials for medical applications .
Biocompatibility and Hemocompatibility
Case Studies:
- A pilot study assessed the biocompatibility of PNVF in vivo, indicating low toxicity and acceptable hemodynamic profiles. This suggests that NVF-derived polymers could be utilized in drug delivery systems and tissue engineering applications without adverse effects on biological systems .
- Further investigations into the blood compatibility of PVAm microparticles demonstrated favorable interactions with red blood cells, making them suitable candidates for applications in blood-contacting devices .
Applications in Drug Delivery and Tissue Engineering
Injectable Hydrogels : Recent studies have explored the use of NVF-based hydrogels for drug delivery systems. These hydrogels can be engineered to respond to physiological conditions, such as pH changes, allowing for controlled release of therapeutic agents. The incorporation of NVF microgels into composite hydrogels has shown promising results in enhancing mechanical properties while maintaining biocompatibility .
Summary of Biological Activities
Properties
IUPAC Name |
N-ethenylformamide | |
---|---|---|
Source | PubChem | |
URL | https://pubchem.ncbi.nlm.nih.gov | |
Description | Data deposited in or computed by PubChem | |
InChI |
InChI=1S/C3H5NO/c1-2-4-3-5/h2-3H,1H2,(H,4,5) | |
Source | PubChem | |
URL | https://pubchem.ncbi.nlm.nih.gov | |
Description | Data deposited in or computed by PubChem | |
InChI Key |
ZQXSMRAEXCEDJD-UHFFFAOYSA-N | |
Source | PubChem | |
URL | https://pubchem.ncbi.nlm.nih.gov | |
Description | Data deposited in or computed by PubChem | |
Canonical SMILES |
C=CNC=O | |
Source | PubChem | |
URL | https://pubchem.ncbi.nlm.nih.gov | |
Description | Data deposited in or computed by PubChem | |
Molecular Formula |
C3H5NO | |
Source | PubChem | |
URL | https://pubchem.ncbi.nlm.nih.gov | |
Description | Data deposited in or computed by PubChem | |
Related CAS |
72018-12-3 | |
Record name | Poly(N-vinylformamide) | |
Source | CAS Common Chemistry | |
URL | https://commonchemistry.cas.org/detail?cas_rn=72018-12-3 | |
Description | CAS Common Chemistry is an open community resource for accessing chemical information. Nearly 500,000 chemical substances from CAS REGISTRY cover areas of community interest, including common and frequently regulated chemicals, and those relevant to high school and undergraduate chemistry classes. This chemical information, curated by our expert scientists, is provided in alignment with our mission as a division of the American Chemical Society. | |
Explanation | The data from CAS Common Chemistry is provided under a CC-BY-NC 4.0 license, unless otherwise stated. | |
DSSTOX Substance ID |
DTXSID9065366 | |
Record name | Formamide, N-ethenyl- | |
Source | EPA DSSTox | |
URL | https://comptox.epa.gov/dashboard/DTXSID9065366 | |
Description | DSSTox provides a high quality public chemistry resource for supporting improved predictive toxicology. | |
Molecular Weight |
71.08 g/mol | |
Source | PubChem | |
URL | https://pubchem.ncbi.nlm.nih.gov | |
Description | Data deposited in or computed by PubChem | |
Physical Description |
Liquid | |
Record name | Formamide, N-ethenyl- | |
Source | EPA Chemicals under the TSCA | |
URL | https://www.epa.gov/chemicals-under-tsca | |
Description | EPA Chemicals under the Toxic Substances Control Act (TSCA) collection contains information on chemicals and their regulations under TSCA, including non-confidential content from the TSCA Chemical Substance Inventory and Chemical Data Reporting. | |
CAS No. |
13162-05-5 | |
Record name | N-Vinylformamide | |
Source | CAS Common Chemistry | |
URL | https://commonchemistry.cas.org/detail?cas_rn=13162-05-5 | |
Description | CAS Common Chemistry is an open community resource for accessing chemical information. Nearly 500,000 chemical substances from CAS REGISTRY cover areas of community interest, including common and frequently regulated chemicals, and those relevant to high school and undergraduate chemistry classes. This chemical information, curated by our expert scientists, is provided in alignment with our mission as a division of the American Chemical Society. | |
Explanation | The data from CAS Common Chemistry is provided under a CC-BY-NC 4.0 license, unless otherwise stated. | |
Record name | N-Vinylformamide | |
Source | ChemIDplus | |
URL | https://pubchem.ncbi.nlm.nih.gov/substance/?source=chemidplus&sourceid=0013162055 | |
Description | ChemIDplus is a free, web search system that provides access to the structure and nomenclature authority files used for the identification of chemical substances cited in National Library of Medicine (NLM) databases, including the TOXNET system. | |
Record name | Formamide, N-ethenyl- | |
Source | EPA Chemicals under the TSCA | |
URL | https://www.epa.gov/chemicals-under-tsca | |
Description | EPA Chemicals under the Toxic Substances Control Act (TSCA) collection contains information on chemicals and their regulations under TSCA, including non-confidential content from the TSCA Chemical Substance Inventory and Chemical Data Reporting. | |
Record name | Formamide, N-ethenyl- | |
Source | EPA DSSTox | |
URL | https://comptox.epa.gov/dashboard/DTXSID9065366 | |
Description | DSSTox provides a high quality public chemistry resource for supporting improved predictive toxicology. | |
Record name | N-vinylformamide | |
Source | European Chemicals Agency (ECHA) | |
URL | https://echa.europa.eu/substance-information/-/substanceinfo/100.032.806 | |
Description | The European Chemicals Agency (ECHA) is an agency of the European Union which is the driving force among regulatory authorities in implementing the EU's groundbreaking chemicals legislation for the benefit of human health and the environment as well as for innovation and competitiveness. | |
Explanation | Use of the information, documents and data from the ECHA website is subject to the terms and conditions of this Legal Notice, and subject to other binding limitations provided for under applicable law, the information, documents and data made available on the ECHA website may be reproduced, distributed and/or used, totally or in part, for non-commercial purposes provided that ECHA is acknowledged as the source: "Source: European Chemicals Agency, http://echa.europa.eu/". Such acknowledgement must be included in each copy of the material. ECHA permits and encourages organisations and individuals to create links to the ECHA website under the following cumulative conditions: Links can only be made to webpages that provide a link to the Legal Notice page. | |
Record name | N-VINYLFORMAMIDE | |
Source | FDA Global Substance Registration System (GSRS) | |
URL | https://gsrs.ncats.nih.gov/ginas/app/beta/substances/14540119E3 | |
Description | The FDA Global Substance Registration System (GSRS) enables the efficient and accurate exchange of information on what substances are in regulated products. Instead of relying on names, which vary across regulatory domains, countries, and regions, the GSRS knowledge base makes it possible for substances to be defined by standardized, scientific descriptions. | |
Explanation | Unless otherwise noted, the contents of the FDA website (www.fda.gov), both text and graphics, are not copyrighted. They are in the public domain and may be republished, reprinted and otherwise used freely by anyone without the need to obtain permission from FDA. Credit to the U.S. Food and Drug Administration as the source is appreciated but not required. | |
Synthesis routes and methods
Procedure details
Retrosynthesis Analysis
AI-Powered Synthesis Planning: Our tool employs the Template_relevance Pistachio, Template_relevance Bkms_metabolic, Template_relevance Pistachio_ringbreaker, Template_relevance Reaxys, Template_relevance Reaxys_biocatalysis model, leveraging a vast database of chemical reactions to predict feasible synthetic routes.
One-Step Synthesis Focus: Specifically designed for one-step synthesis, it provides concise and direct routes for your target compounds, streamlining the synthesis process.
Accurate Predictions: Utilizing the extensive PISTACHIO, BKMS_METABOLIC, PISTACHIO_RINGBREAKER, REAXYS, REAXYS_BIOCATALYSIS database, our tool offers high-accuracy predictions, reflecting the latest in chemical research and data.
Strategy Settings
Precursor scoring | Relevance Heuristic |
---|---|
Min. plausibility | 0.01 |
Model | Template_relevance |
Template Set | Pistachio/Bkms_metabolic/Pistachio_ringbreaker/Reaxys/Reaxys_biocatalysis |
Top-N result to add to graph | 6 |
Feasible Synthetic Routes
Disclaimer and Information on In-Vitro Research Products
Please be aware that all articles and product information presented on BenchChem are intended solely for informational purposes. The products available for purchase on BenchChem are specifically designed for in-vitro studies, which are conducted outside of living organisms. In-vitro studies, derived from the Latin term "in glass," involve experiments performed in controlled laboratory settings using cells or tissues. It is important to note that these products are not categorized as medicines or drugs, and they have not received approval from the FDA for the prevention, treatment, or cure of any medical condition, ailment, or disease. We must emphasize that any form of bodily introduction of these products into humans or animals is strictly prohibited by law. It is essential to adhere to these guidelines to ensure compliance with legal and ethical standards in research and experimentation.