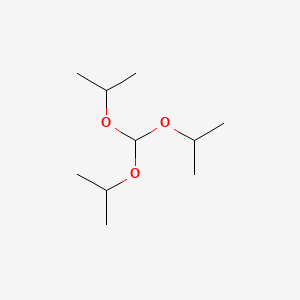
Triisopropyl orthoformate
Overview
Description
Triisopropyl orthoformate is an organic compound with the molecular formula C10H22O3. It is a colorless liquid with a pungent odor and is primarily used as a reagent in organic synthesis. This compound is particularly valuable in the protection of alcohols and amines, making it a versatile tool in various chemical reactions .
Scientific Research Applications
Triisopropyl orthoformate has a wide range of applications in scientific research:
Chemistry: It is used as a reagent in organic synthesis for the protection of functional groups and as a precursor for the synthesis of other compounds.
Biology: this compound is employed in the modification of biomolecules, such as the protection of hydroxyl groups in nucleotides and carbohydrates.
Medicine: It is used in the synthesis of pharmaceutical intermediates and active pharmaceutical ingredients.
Industry: this compound is utilized in the production of specialty chemicals and materials
Safety and Hazards
Triisopropyl Orthoformate is considered hazardous. It is flammable and can cause skin irritation, serious eye irritation, and respiratory irritation . It is recommended to avoid breathing its vapors and to use personal protective equipment when handling it .
Relevant Papers The most relevant paper retrieved is “Applications of alkyl orthoesters as valuable substrates in organic transformations, focusing on reaction media” published in RSC Advances . This paper provides a comprehensive review of the applications of alkyl orthoesters, including this compound, in various organic reactions.
Mechanism of Action
Target of Action
Triisopropyl orthoformate (TIPO) is a chemical compound used as a reagent in organic synthesis . It doesn’t have a specific biological target, but rather, it interacts with other chemical substances during the synthesis process.
Mode of Action
The mode of action of TIPO is primarily through its role as a reagent in organic synthesis. It is formed during the reaction of dichlorofluoromethane with potassium isopropoxide in isopropyl alcohol . It can be used to prepare other compounds, such as 2-(diisopropoxymethyl)oxirane .
Result of Action
The result of TIPO’s action is the formation of new chemical compounds. For example, it can be used for the preparation of 2-(diisopropoxymethyl)oxirane . The exact molecular and cellular effects would depend on the specific reactions it is used in.
Preparation Methods
Synthetic Routes and Reaction Conditions: Triisopropyl orthoformate is typically synthesized through the reaction of dichlorofluoromethane with potassium isopropoxide in isopropyl alcohol. The reaction proceeds as follows: [ \text{CHClF}_2 + 3 \text{KOCH(CH}_3\text{)}_2 \rightarrow \text{C[OCH(CH}_3\text{)}_2]_3 + 3 \text{KCl} + \text{KF} ] The reaction is carried out under controlled conditions to ensure the purity and yield of the product .
Industrial Production Methods: In industrial settings, the production of this compound involves similar synthetic routes but on a larger scale. The reaction conditions are optimized to maximize yield and minimize impurities. The separation of this compound from the reaction mixture can be achieved using techniques such as distillation and pervaporation through dense carbon nanotube membranes .
Chemical Reactions Analysis
Types of Reactions: Triisopropyl orthoformate undergoes various chemical reactions, including:
Hydrolysis: In the presence of water, this compound hydrolyzes to form isopropyl alcohol and formic acid.
Protection Reactions: It is commonly used to protect alcohols and amines by forming stable orthoformate derivatives.
Substitution Reactions: this compound can participate in nucleophilic substitution reactions, where the isopropoxy groups are replaced by other nucleophiles.
Common Reagents and Conditions:
Hydrolysis: Water or aqueous acid solutions.
Protection Reactions: Alcohols or amines in the presence of an acid catalyst.
Substitution Reactions: Various nucleophiles such as halides, thiols, and amines.
Major Products:
Hydrolysis: Isopropyl alcohol and formic acid.
Protection Reactions: Orthoformate derivatives of alcohols and amines.
Substitution Reactions: Substituted orthoformates.
Comparison with Similar Compounds
Triisopropyl orthoformate can be compared with other similar compounds such as:
Trimethyl orthoformate: A simpler orthoester with three methoxy groups. It is less sterically hindered and more reactive than this compound.
Triethyl orthoformate: Contains three ethoxy groups and is used in similar applications but has different reactivity and steric properties.
Triethyl orthoacetate: An orthoester with an acetyl group, used in different synthetic applications due to its unique reactivity.
Uniqueness: this compound is unique due to its steric bulk and the stability of its orthoformate derivatives. This makes it particularly useful in reactions where steric hindrance is beneficial, such as in the protection of sensitive functional groups .
Properties
IUPAC Name |
2-[di(propan-2-yloxy)methoxy]propane | |
---|---|---|
Source | PubChem | |
URL | https://pubchem.ncbi.nlm.nih.gov | |
Description | Data deposited in or computed by PubChem | |
InChI |
InChI=1S/C10H22O3/c1-7(2)11-10(12-8(3)4)13-9(5)6/h7-10H,1-6H3 | |
Source | PubChem | |
URL | https://pubchem.ncbi.nlm.nih.gov | |
Description | Data deposited in or computed by PubChem | |
InChI Key |
FPIVAWNGRDHRSQ-UHFFFAOYSA-N | |
Source | PubChem | |
URL | https://pubchem.ncbi.nlm.nih.gov | |
Description | Data deposited in or computed by PubChem | |
Canonical SMILES |
CC(C)OC(OC(C)C)OC(C)C | |
Source | PubChem | |
URL | https://pubchem.ncbi.nlm.nih.gov | |
Description | Data deposited in or computed by PubChem | |
Molecular Formula |
C10H22O3 | |
Source | PubChem | |
URL | https://pubchem.ncbi.nlm.nih.gov | |
Description | Data deposited in or computed by PubChem | |
DSSTOX Substance ID |
DTXSID7063479 | |
Record name | Propane, 2,2',2''-[methylidynetris(oxy)]tris- | |
Source | EPA DSSTox | |
URL | https://comptox.epa.gov/dashboard/DTXSID7063479 | |
Description | DSSTox provides a high quality public chemistry resource for supporting improved predictive toxicology. | |
Molecular Weight |
190.28 g/mol | |
Source | PubChem | |
URL | https://pubchem.ncbi.nlm.nih.gov | |
Description | Data deposited in or computed by PubChem | |
CAS No. |
4447-60-3 | |
Record name | 2,2′,2′′-[Methylidynetris(oxy)]tris[propane] | |
Source | CAS Common Chemistry | |
URL | https://commonchemistry.cas.org/detail?cas_rn=4447-60-3 | |
Description | CAS Common Chemistry is an open community resource for accessing chemical information. Nearly 500,000 chemical substances from CAS REGISTRY cover areas of community interest, including common and frequently regulated chemicals, and those relevant to high school and undergraduate chemistry classes. This chemical information, curated by our expert scientists, is provided in alignment with our mission as a division of the American Chemical Society. | |
Explanation | The data from CAS Common Chemistry is provided under a CC-BY-NC 4.0 license, unless otherwise stated. | |
Record name | Propane, 2,2',2''-(methylidynetris(oxy))tris- | |
Source | ChemIDplus | |
URL | https://pubchem.ncbi.nlm.nih.gov/substance/?source=chemidplus&sourceid=0004447603 | |
Description | ChemIDplus is a free, web search system that provides access to the structure and nomenclature authority files used for the identification of chemical substances cited in National Library of Medicine (NLM) databases, including the TOXNET system. | |
Record name | Propane, 2,2',2''-[methylidynetris(oxy)]tris- | |
Source | EPA Chemicals under the TSCA | |
URL | https://www.epa.gov/chemicals-under-tsca | |
Description | EPA Chemicals under the Toxic Substances Control Act (TSCA) collection contains information on chemicals and their regulations under TSCA, including non-confidential content from the TSCA Chemical Substance Inventory and Chemical Data Reporting. | |
Record name | Propane, 2,2',2''-[methylidynetris(oxy)]tris- | |
Source | EPA DSSTox | |
URL | https://comptox.epa.gov/dashboard/DTXSID7063479 | |
Description | DSSTox provides a high quality public chemistry resource for supporting improved predictive toxicology. | |
Record name | 2,2',2''-[methylidynetris(oxy)]trispropane | |
Source | European Chemicals Agency (ECHA) | |
URL | https://echa.europa.eu/substance-information/-/substanceinfo/100.022.445 | |
Description | The European Chemicals Agency (ECHA) is an agency of the European Union which is the driving force among regulatory authorities in implementing the EU's groundbreaking chemicals legislation for the benefit of human health and the environment as well as for innovation and competitiveness. | |
Explanation | Use of the information, documents and data from the ECHA website is subject to the terms and conditions of this Legal Notice, and subject to other binding limitations provided for under applicable law, the information, documents and data made available on the ECHA website may be reproduced, distributed and/or used, totally or in part, for non-commercial purposes provided that ECHA is acknowledged as the source: "Source: European Chemicals Agency, http://echa.europa.eu/". Such acknowledgement must be included in each copy of the material. ECHA permits and encourages organisations and individuals to create links to the ECHA website under the following cumulative conditions: Links can only be made to webpages that provide a link to the Legal Notice page. | |
Retrosynthesis Analysis
AI-Powered Synthesis Planning: Our tool employs the Template_relevance Pistachio, Template_relevance Bkms_metabolic, Template_relevance Pistachio_ringbreaker, Template_relevance Reaxys, Template_relevance Reaxys_biocatalysis model, leveraging a vast database of chemical reactions to predict feasible synthetic routes.
One-Step Synthesis Focus: Specifically designed for one-step synthesis, it provides concise and direct routes for your target compounds, streamlining the synthesis process.
Accurate Predictions: Utilizing the extensive PISTACHIO, BKMS_METABOLIC, PISTACHIO_RINGBREAKER, REAXYS, REAXYS_BIOCATALYSIS database, our tool offers high-accuracy predictions, reflecting the latest in chemical research and data.
Strategy Settings
Precursor scoring | Relevance Heuristic |
---|---|
Min. plausibility | 0.01 |
Model | Template_relevance |
Template Set | Pistachio/Bkms_metabolic/Pistachio_ringbreaker/Reaxys/Reaxys_biocatalysis |
Top-N result to add to graph | 6 |
Feasible Synthetic Routes
Q1: What is Triisopropyl Orthoformate primarily used for in chemical synthesis?
A1: this compound (TIPOF) serves as a versatile reagent in organic synthesis, particularly for protecting hydroxyl groups and forming acetals. For instance, it facilitates the conversion of hydroxyacetophenones into their corresponding ethylene or trimethylene acetals. [, ] Additionally, TIPOF acts as a dehydrating agent, enabling the synthesis of aryl ethers through the reaction of cyclohexanones with alcohols. []
Q2: Can you provide the structural characterization of this compound?
A2: this compound has the molecular formula C10H22O3 and a molecular weight of 190.28 g/mol. While the provided abstracts do not offer detailed spectroscopic data, its 1H and 13C NMR spectra would show characteristic peaks for the isopropyl groups and the central carbon atom bonded to three oxygen atoms.
Q3: How does this compound contribute to the synthesis of aryl ethers from cyclohexanones?
A3: In the presence of a Palladium catalyst, TIPOF participates in a multi-step process to produce aryl ethers from cyclohexanones. Firstly, the Pd catalyst activates the C-H bond in cyclohexanones. Subsequently, TIPOF acts as a nucleophile, enabling the addition and elimination reactions necessary to form the aryl ether. Its role as a dehydrating agent further drives the reaction towards the desired product. []
Q4: Beyond aryl ether synthesis, are there other applications for this compound in organic reactions?
A4: Yes, TIPOF plays a crucial role in the synthesis of vertically aligned carbon nanotube (VA-CNT) membranes. During the membrane fabrication process, TIPOF, along with n-hexane, is used to assess the membrane's separation capabilities. The ability of the VA-CNT membrane to separate the larger TIPOF molecule from the smaller n-hexane molecule demonstrates its potential for separating liquid mixtures. []
Q5: Are there any safety concerns associated with handling this compound?
A5: While specific safety hazards for TIPOF were not mentioned in the provided abstracts, it's essential to handle all chemicals with caution. Standard laboratory safety practices, including wearing gloves, safety glasses, and a lab coat, should be followed. [] Storing TIPOF in a dry environment is also recommended due to its sensitivity to moisture. []
Disclaimer and Information on In-Vitro Research Products
Please be aware that all articles and product information presented on BenchChem are intended solely for informational purposes. The products available for purchase on BenchChem are specifically designed for in-vitro studies, which are conducted outside of living organisms. In-vitro studies, derived from the Latin term "in glass," involve experiments performed in controlled laboratory settings using cells or tissues. It is important to note that these products are not categorized as medicines or drugs, and they have not received approval from the FDA for the prevention, treatment, or cure of any medical condition, ailment, or disease. We must emphasize that any form of bodily introduction of these products into humans or animals is strictly prohibited by law. It is essential to adhere to these guidelines to ensure compliance with legal and ethical standards in research and experimentation.