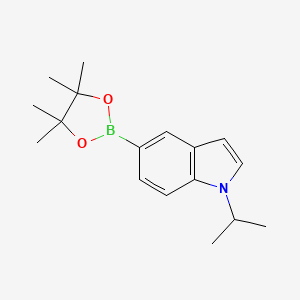
1-(propan-2-yl)-5-(tetramethyl-1,3,2-dioxaborolan-2-yl)-1H-indole
- Click on QUICK INQUIRY to receive a quote from our team of experts.
- With the quality product at a COMPETITIVE price, you can focus more on your research.
Overview
Description
1-(Propan-2-yl)-5-(tetramethyl-1,3,2-dioxaborolan-2-yl)-1H-indole is a chemical compound that has garnered attention in various fields of scientific research. This compound is characterized by its unique structure, which includes an indole core substituted with a propan-2-yl group and a tetramethyl-1,3,2-dioxaborolan-2-yl group. The presence of these functional groups imparts distinct chemical properties to the compound, making it valuable for various applications.
Preparation Methods
The synthesis of 1-(propan-2-yl)-5-(tetramethyl-1,3,2-dioxaborolan-2-yl)-1H-indole typically involves several steps. One common synthetic route includes the following steps:
Formation of the Indole Core: The indole core can be synthesized through a Fischer indole synthesis, which involves the reaction of phenylhydrazine with a ketone or aldehyde under acidic conditions.
Introduction of the Propan-2-yl Group: The propan-2-yl group can be introduced through alkylation reactions using appropriate alkyl halides and bases.
Attachment of the Tetramethyl-1,3,2-dioxaborolan-2-yl Group: This step often involves the use of boronic acid derivatives and palladium-catalyzed cross-coupling reactions, such as the Suzuki-Miyaura coupling, to attach the boron-containing group to the indole core.
Industrial production methods for this compound may involve optimization of these synthetic routes to enhance yield and purity while minimizing costs and environmental impact.
Chemical Reactions Analysis
1-(Propan-2-yl)-5-(tetramethyl-1,3,2-dioxaborolan-2-yl)-1H-indole undergoes various chemical reactions, including:
Oxidation: The compound can be oxidized using oxidizing agents such as hydrogen peroxide or potassium permanganate, leading to the formation of corresponding oxidized products.
Reduction: Reduction reactions can be carried out using reducing agents like sodium borohydride or lithium aluminum hydride to yield reduced derivatives.
Substitution: The compound can undergo substitution reactions, particularly at the boron-containing group, using nucleophiles or electrophiles under appropriate conditions.
Common reagents and conditions used in these reactions include palladium catalysts for cross-coupling reactions, strong acids or bases for substitution reactions, and specific oxidizing or reducing agents for redox reactions. The major products formed from these reactions depend on the specific reagents and conditions employed.
Scientific Research Applications
1-(Propan-2-yl)-5-(tetramethyl-1,3,2-dioxaborolan-2-yl)-1H-indole has a wide range of scientific research applications, including:
Chemistry: The compound is used as a building block in organic synthesis, particularly in the development of new pharmaceuticals and agrochemicals.
Biology: It is employed in the study of biological processes and pathways, often as a probe or ligand in biochemical assays.
Medicine: The compound has potential therapeutic applications, including as an anti-tubercular agent and in the treatment of other diseases.
Industry: It is used in the production of advanced materials and as a catalyst in various industrial processes.
Mechanism of Action
The mechanism of action of 1-(propan-2-yl)-5-(tetramethyl-1,3,2-dioxaborolan-2-yl)-1H-indole involves its interaction with specific molecular targets and pathways. The compound can act as an inhibitor or activator of certain enzymes, receptors, or other biomolecules, leading to modulation of biological processes. The exact molecular targets and pathways involved depend on the specific application and context in which the compound is used.
Comparison with Similar Compounds
1-(Propan-2-yl)-5-(tetramethyl-1,3,2-dioxaborolan-2-yl)-1H-indole can be compared with other similar compounds, such as:
1-(Propan-2-yl)-1H-indole: Lacks the boron-containing group, resulting in different chemical properties and reactivity.
5-(Tetramethyl-1,3,2-dioxaborolan-2-yl)-1H-indole: Lacks the propan-2-yl group, affecting its biological activity and applications.
1-(Propan-2-yl)-5-bromo-1H-indole:
The uniqueness of this compound lies in its combination of functional groups, which imparts distinct chemical and biological properties, making it valuable for a wide range of applications.
Biological Activity
The compound 1-(propan-2-yl)-5-(tetramethyl-1,3,2-dioxaborolan-2-yl)-1H-indole is a complex organic molecule that integrates an indole structure with a boronate moiety. This unique combination endows it with significant biological activity, particularly in medicinal chemistry and drug development. The molecular formula for this compound is C14H21BNO2, with a molecular weight of approximately 257.14 g/mol .
Biological Activity Overview
Indole derivatives, including this compound, have been extensively studied for their diverse biological activities. These activities include:
- Anticancer properties : Many indole derivatives exhibit potent growth inhibition against various cancer cell lines.
- Antimicrobial effects : Some studies suggest that indole derivatives possess significant antibacterial and antifungal properties.
- Neuroprotective effects : Certain indole compounds have shown promise in protecting neuronal cells from oxidative stress.
The biological activity of this compound is believed to stem from its ability to interact with various biological targets:
- Inhibition of key enzymes : The compound may inhibit critical enzymes involved in cancer cell proliferation.
- Modulation of signaling pathways : It can affect cellular signaling pathways that regulate cell growth and apoptosis.
- Reactive oxygen species (ROS) management : The compound may help in reducing oxidative stress within cells.
Synthesis Methods
The synthesis of this compound can be achieved through various methods, including:
- Boronate esterification : This involves the reaction of indole with boronic acid derivatives under acidic conditions.
- Cross-coupling reactions : Utilizing palladium or nickel catalysts to facilitate the formation of carbon-boron bonds.
Structural Features
The presence of both the propan-2-yl and dioxaborolane groups enhances the stability and reactivity of this compound compared to other similar indole derivatives. Below is a comparison table highlighting structural similarities and differences with other compounds:
Compound Name | Molecular Formula | Unique Features |
---|---|---|
5-Indoleboronic Acid Pinacol Ester | C14H18BNO2 | Lacks propan-2-yl group; simpler structure |
tert-butyl 5-chloroindole | C13H14ClN | Contains chlorine; different reactivity profile |
4-(4,4,5,5-tetramethyl-1,3,2-dioxaborolan-2-yl)indoline | C19H26BNO4 | More complex structure with additional functional groups |
5-Iodoindole | C8H6N | Halogenated variant; different reactivity and biological activity |
Anticancer Activity
A study investigating the anticancer properties of various indole derivatives highlighted that compounds similar to this compound exhibited selective toxicity towards tumorigenic cells while sparing non-tumorigenic cells at concentrations as low as 10 µM . The mechanism was linked to the modulation of apoptosis-related signaling pathways.
Antimicrobial Properties
Research has also demonstrated that certain indole derivatives possess antimicrobial activity against both Gram-positive and Gram-negative bacteria. The mechanism involves disruption of bacterial cell membranes and inhibition of essential metabolic pathways .
Neuroprotective Effects
In neuropharmacological studies, indole derivatives were shown to protect neuronal cells from oxidative damage induced by neurotoxic agents. This protective effect is attributed to their ability to scavenge free radicals and modulate inflammatory responses .
Properties
Molecular Formula |
C17H24BNO2 |
---|---|
Molecular Weight |
285.2 g/mol |
IUPAC Name |
1-propan-2-yl-5-(4,4,5,5-tetramethyl-1,3,2-dioxaborolan-2-yl)indole |
InChI |
InChI=1S/C17H24BNO2/c1-12(2)19-10-9-13-11-14(7-8-15(13)19)18-20-16(3,4)17(5,6)21-18/h7-12H,1-6H3 |
InChI Key |
LDQCCMBWNAHYQG-UHFFFAOYSA-N |
Canonical SMILES |
B1(OC(C(O1)(C)C)(C)C)C2=CC3=C(C=C2)N(C=C3)C(C)C |
Origin of Product |
United States |
Disclaimer and Information on In-Vitro Research Products
Please be aware that all articles and product information presented on BenchChem are intended solely for informational purposes. The products available for purchase on BenchChem are specifically designed for in-vitro studies, which are conducted outside of living organisms. In-vitro studies, derived from the Latin term "in glass," involve experiments performed in controlled laboratory settings using cells or tissues. It is important to note that these products are not categorized as medicines or drugs, and they have not received approval from the FDA for the prevention, treatment, or cure of any medical condition, ailment, or disease. We must emphasize that any form of bodily introduction of these products into humans or animals is strictly prohibited by law. It is essential to adhere to these guidelines to ensure compliance with legal and ethical standards in research and experimentation.