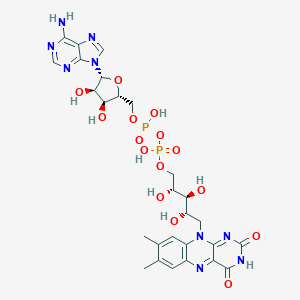
Flavin adenine dinucleotide
Overview
Description
Flavin adenine dinucleotide is a redox-active coenzyme associated with various proteins and involved in several enzymatic reactions in metabolism. It is a derivative of riboflavin (vitamin B2) and plays a crucial role in biological oxidation-reduction reactions. This compound can exist in multiple redox states, including the fully oxidized quinone form, the semiquinone form, and the fully reduced hydroquinone form .
Mechanism of Action
Target of Action
Flavin Adenine Dinucleotide (FAD) is a redox-active coenzyme associated with various proteins . It is the ubiquitous cofactor of a number of flavoenzymes, most of which are involved in redox reactions . Some of the primary targets of FAD include NADH dehydrogenase , p-hydroxybenzoate hydroxylase , Nitric oxide synthase, brain , and NAD (P)H dehydrogenase .
Mode of Action
FAD can exist in four redox states, which are the flavin-N (5)-oxide, quinone, semiquinone, and hydroquinone . FAD is converted between these states by accepting or donating electrons . In its fully oxidized form, or quinone form, FAD accepts two electrons and two protons to become FADH2 (hydroquinone form) . The semiquinone (FADH) can be formed by either reduction of FAD or oxidation of FADH2 by accepting or donating one electron and one proton, respectively .
Biochemical Pathways
FAD is involved in several enzymatic reactions in metabolism . It plays a crucial role in the electron transport chain where it is involved in the first enzyme complex 1 . A FMN (flavin adenine mononucleotide) as an oxidizing agent is used to react with NADH for the second step in the electron transport chain .
Pharmacokinetics
The pharmacokinetics of FAD have been studied in rats . After the intravenous bolus administration of a single dose of FMN (500nmol/kg), FAD was identified in plasma . Increased levels of fad in plasma were much less pronounced than that of rf levels .
Result of Action
FAD is involved in several cellular processes such as metabolism, bioenergetics, protein folding, ROS production, as well as defense against oxidative stress, redox epigenetics, cell differentiation, and many others . Therefore, a fine coordination among apo-flavoprotein turn-over, cofactor availability, and, in eukaryotic cells, sub-cellular flavin trafficking, is required in order to ensure correct flavoproteome maintenance .
Action Environment
The action of FAD is influenced by environmental factors such as the availability of riboflavin (Rf; vitamin B2), which is essential for the biosynthesis of FAD . The biosynthesis of FAD, starting from extracellular Rf, requires the sequential action of specialized transporters (RFVTs), riboflavin kinase (RFK), which catalyze the formation of FMN, and FAD synthase or FMN adenylyl transferase (FADS or FMNAT), which adenylates FMN to FAD .
Biochemical Analysis
Biochemical Properties
Flavin adenine dinucleotide plays a vital role in biochemical reactions. It is associated with various proteins and is involved in several enzymatic reactions in metabolism . This compound can exist in four redox states, which are the flavin-N (5)-oxide, quinone, semiquinone, and hydroquinone . It is converted between these states by accepting or donating electrons .
Cellular Effects
This compound is involved in numerous important metabolic pathways where the biological function is intrinsically related to its transient conformations . Several cellular processes depend on this compound, namely: metabolism, bioenergetics, protein folding, ROS production, as well as defense against oxidative stress, redox epigenetics, cell differentiation, and many others .
Molecular Mechanism
This compound, in its fully oxidized form, or quinone form, accepts two electrons and two protons to become FADH2 (hydroquinone form) . The semiquinone (FADH·) can be formed by either reduction of this compound or oxidation of FADH2 by accepting or donating one electron and one proton, respectively .
Temporal Effects in Laboratory Settings
In laboratory settings, this compound exhibits transient conformations . These transient conformations are crucial for its function in biochemical reactions . The stability and degradation of this compound over time in laboratory settings can affect its function and the results of biochemical reactions .
Dosage Effects in Animal Models
The effects of this compound vary with different dosages in animal models . High doses of this compound can induce renal disease in the organism . A physiologic dose of this compound is beneficial in terms of biological activity .
Metabolic Pathways
This compound is involved in several enzymatic reactions in metabolism . It is a crucial part of the electron transport chain, where it acts as a cofactor in redox reactions . It is also involved in the first enzyme complex 1 of the electron transport chain .
Transport and Distribution
This compound is transported and distributed within cells and tissues through specialized transporters . It is essential for the correct maintenance of the flavoproteome .
Subcellular Localization
The subcellular localization of this compound is crucial for its function. It is involved in numerous important metabolic pathways where its biological function is intrinsically related to its transient conformations . The confined space of enzymes requires this compound set in its specific intermediate conformation .
Preparation Methods
Synthetic Routes and Reaction Conditions: Flavin adenine dinucleotide can be synthesized enzymatically from riboflavin through a series of phosphorylation and adenylylation reactions. The key enzymes involved are riboflavin kinase, which converts riboflavin to flavin mononucleotide, and flavin mononucleotide adenylyltransferase, which converts flavin mononucleotide to this compound .
Industrial Production Methods: Industrial production of this compound typically involves microbial fermentation processes using genetically engineered strains of bacteria or yeast that overexpress the necessary enzymes. These microorganisms are cultured in bioreactors under controlled conditions to optimize the yield of this compound .
Chemical Reactions Analysis
Types of Reactions: Flavin adenine dinucleotide undergoes various types of reactions, primarily oxidation and reduction. It can accept or donate electrons and protons, transitioning between its different redox states. The fully oxidized form (quinone) can accept two electrons and two protons to become the fully reduced form (hydroquinone). The semiquinone form can be formed by either reduction of the quinone form or oxidation of the hydroquinone form .
Common Reagents and Conditions: Common reagents used in reactions involving this compound include molecular oxygen, hydrogen peroxide, and various organic and inorganic substrates. The reactions typically occur under physiological conditions, such as neutral pH and moderate temperatures .
Major Products: The major products formed from reactions involving this compound depend on the specific enzymatic context. For example, in the electron transport chain, this compound is involved in the production of adenosine triphosphate through oxidative phosphorylation .
Scientific Research Applications
Flavin adenine dinucleotide has a wide range of scientific research applications:
Chemistry: It is used as a cofactor in various redox reactions and as a model compound for studying electron transfer processes.
Biology: this compound is essential for the function of many flavoproteins involved in cellular respiration, fatty acid oxidation, and amino acid metabolism.
Medicine: It is studied for its role in metabolic disorders and mitochondrial diseases. This compound analogs are being explored as potential therapeutic agents.
Industry: this compound is used in biosensors and bioelectronic devices due to its redox properties.
Comparison with Similar Compounds
Flavin mononucleotide: Another derivative of riboflavin, flavin mononucleotide is also a redox-active coenzyme but lacks the adenine moiety present in flavin adenine dinucleotide.
Nicotinamide adenine dinucleotide: This coenzyme is involved in redox reactions but contains nicotinamide instead of flavin.
Nicotinamide adenine dinucleotide phosphate: Similar to nicotinamide adenine dinucleotide but with an additional phosphate group, it is involved in anabolic reactions.
Uniqueness: this compound is unique in its ability to participate in both one-electron and two-electron transfer processes, making it a versatile coenzyme in various biochemical pathways. Its role in bridging the gap between two-electron and one-electron processes is critical for maintaining cellular redox balance .
Properties
IUPAC Name |
[[5-(6-aminopurin-9-yl)-3,4-dihydroxyoxolan-2-yl]methoxy-hydroxyphosphoryl] [5-(7,8-dimethyl-2,4-dioxobenzo[g]pteridin-10-yl)-2,3,4-trihydroxypentyl] hydrogen phosphate | |
---|---|---|
Details | Computed by Lexichem TK 2.7.0 (PubChem release 2021.05.07) | |
Source | PubChem | |
URL | https://pubchem.ncbi.nlm.nih.gov | |
Description | Data deposited in or computed by PubChem | |
InChI |
InChI=1S/C27H33N9O15P2/c1-10-3-12-13(4-11(10)2)35(24-18(32-12)25(42)34-27(43)33-24)5-14(37)19(39)15(38)6-48-52(44,45)51-53(46,47)49-7-16-20(40)21(41)26(50-16)36-9-31-17-22(28)29-8-30-23(17)36/h3-4,8-9,14-16,19-21,26,37-41H,5-7H2,1-2H3,(H,44,45)(H,46,47)(H2,28,29,30)(H,34,42,43) | |
Details | Computed by InChI 1.0.6 (PubChem release 2021.05.07) | |
Source | PubChem | |
URL | https://pubchem.ncbi.nlm.nih.gov | |
Description | Data deposited in or computed by PubChem | |
InChI Key |
VWWQXMAJTJZDQX-UHFFFAOYSA-N | |
Details | Computed by InChI 1.0.6 (PubChem release 2021.05.07) | |
Source | PubChem | |
URL | https://pubchem.ncbi.nlm.nih.gov | |
Description | Data deposited in or computed by PubChem | |
Canonical SMILES |
CC1=CC2=C(C=C1C)N(C3=NC(=O)NC(=O)C3=N2)CC(C(C(COP(=O)(O)OP(=O)(O)OCC4C(C(C(O4)N5C=NC6=C(N=CN=C65)N)O)O)O)O)O | |
Details | Computed by OEChem 2.3.0 (PubChem release 2021.05.07) | |
Source | PubChem | |
URL | https://pubchem.ncbi.nlm.nih.gov | |
Description | Data deposited in or computed by PubChem | |
Molecular Formula |
C27H33N9O15P2 | |
Details | Computed by PubChem 2.1 (PubChem release 2021.05.07) | |
Source | PubChem | |
URL | https://pubchem.ncbi.nlm.nih.gov | |
Description | Data deposited in or computed by PubChem | |
DSSTOX Substance ID |
DTXSID70859284 | |
Record name | CERAPP_13213 | |
Source | EPA DSSTox | |
URL | https://comptox.epa.gov/dashboard/DTXSID70859284 | |
Description | DSSTox provides a high quality public chemistry resource for supporting improved predictive toxicology. | |
Molecular Weight |
785.5 g/mol | |
Details | Computed by PubChem 2.1 (PubChem release 2021.05.07) | |
Source | PubChem | |
URL | https://pubchem.ncbi.nlm.nih.gov | |
Description | Data deposited in or computed by PubChem | |
CAS No. |
146-14-5 | |
Record name | flavitan | |
Source | DTP/NCI | |
URL | https://dtp.cancer.gov/dtpstandard/servlet/dwindex?searchtype=NSC&outputformat=html&searchlist=112207 | |
Description | The NCI Development Therapeutics Program (DTP) provides services and resources to the academic and private-sector research communities worldwide to facilitate the discovery and development of new cancer therapeutic agents. | |
Explanation | Unless otherwise indicated, all text within NCI products is free of copyright and may be reused without our permission. Credit the National Cancer Institute as the source. | |
Retrosynthesis Analysis
AI-Powered Synthesis Planning: Our tool employs the Template_relevance Pistachio, Template_relevance Bkms_metabolic, Template_relevance Pistachio_ringbreaker, Template_relevance Reaxys, Template_relevance Reaxys_biocatalysis model, leveraging a vast database of chemical reactions to predict feasible synthetic routes.
One-Step Synthesis Focus: Specifically designed for one-step synthesis, it provides concise and direct routes for your target compounds, streamlining the synthesis process.
Accurate Predictions: Utilizing the extensive PISTACHIO, BKMS_METABOLIC, PISTACHIO_RINGBREAKER, REAXYS, REAXYS_BIOCATALYSIS database, our tool offers high-accuracy predictions, reflecting the latest in chemical research and data.
Strategy Settings
Precursor scoring | Relevance Heuristic |
---|---|
Min. plausibility | 0.01 |
Model | Template_relevance |
Template Set | Pistachio/Bkms_metabolic/Pistachio_ringbreaker/Reaxys/Reaxys_biocatalysis |
Top-N result to add to graph | 6 |
Feasible Synthetic Routes
Disclaimer and Information on In-Vitro Research Products
Please be aware that all articles and product information presented on BenchChem are intended solely for informational purposes. The products available for purchase on BenchChem are specifically designed for in-vitro studies, which are conducted outside of living organisms. In-vitro studies, derived from the Latin term "in glass," involve experiments performed in controlled laboratory settings using cells or tissues. It is important to note that these products are not categorized as medicines or drugs, and they have not received approval from the FDA for the prevention, treatment, or cure of any medical condition, ailment, or disease. We must emphasize that any form of bodily introduction of these products into humans or animals is strictly prohibited by law. It is essential to adhere to these guidelines to ensure compliance with legal and ethical standards in research and experimentation.