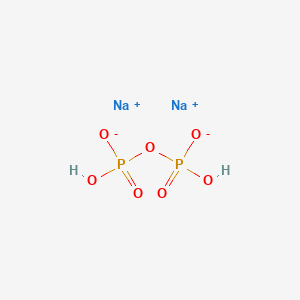
Disodium diphosphate
Overview
Description
Sodium acid pyrophosphate, also known as disodium dihydrogen pyrophosphate, is an inorganic compound with the chemical formula Na₂H₂P₂O₇. It consists of sodium cations and dihydrogen pyrophosphate anions. This white, water-soluble solid is commonly used as a buffering and chelating agent in various applications, particularly in the food industry .
Preparation Methods
Synthetic Routes and Reaction Conditions: Sodium acid pyrophosphate is synthesized by partially neutralizing food-grade phosphoric acid with sodium hydroxide or sodium carbonate to form monosodium phosphate. The monosodium phosphate is then dehydrated at approximately 250°C to form sodium acid pyrophosphate .
Industrial Production Methods: In industrial settings, food-grade soda ash is added to a neutralizer and heated while stirring. Food-grade phosphoric acid is then added for a neutralization reaction, controlling the reaction endpoint pH to 4-4.4 to generate sodium dihydrogen phosphate. The solution is filtered, concentrated by evaporation, cooled to crystallize, centrifuged, and dried to form anhydrous sodium dihydrogen phosphate. This is then heated at 140-200°C to convert it into sodium acid pyrophosphate .
Types of Reactions:
Acid-Base Reactions: Sodium acid pyrophosphate reacts with sodium bicarbonate to release carbon dioxide, which is used in baking as a leavening agent.
Hydrolysis: When exposed to moisture, sodium acid pyrophosphate hydrolyzes to form sodium orthophosphate.
Common Reagents and Conditions:
Sodium Bicarbonate: Used in baking to produce carbon dioxide gas.
Water: Hydrolyzes sodium acid pyrophosphate to sodium orthophosphate.
Major Products:
Carbon Dioxide (CO₂): Produced during the reaction with sodium bicarbonate.
Sodium Orthophosphate (Na₃PO₄): Formed during hydrolysis.
Scientific Research Applications
Sodium acid pyrophosphate has a wide range of applications in scientific research and industry:
Food Industry: Used as a leavening agent in baking powders and as a chelating agent to prevent discoloration in processed potatoes and canned seafood.
Water Treatment: Acts as a water softener and dispersing agent.
Dental Care: Used as a tartar control agent in toothpaste and dental floss.
Cleaning Agents: Employed in some dairy applications for cleaning and in petroleum production as a dispersant in oil well drilling muds.
Mechanism of Action
As a leavening agent, sodium acid pyrophosphate reacts with sodium bicarbonate in the presence of moisture and heat to produce carbon dioxide gas. This gas imparts a light and fluffy texture to baked goods . Additionally, sodium acid pyrophosphate forms ionic bonds with starches and proteins in dough, providing a moist texture .
Comparison with Similar Compounds
- Disodium Phosphate (Na₂HPO₄)
- Tetrasodium Pyrophosphate (Na₄P₂O₇)
- Sodium Hexametaphosphate (Na₆P₆O₁₈)
- Pentasodium Triphosphate (Na₅P₃O₁₀)
Comparison: Sodium acid pyrophosphate is unique in its ability to act as both a leavening agent and a chelating agent. While disodium phosphate and tetrasodium pyrophosphate are also used in food processing, they do not possess the same leavening properties. Sodium hexametaphosphate and pentasodium triphosphate are primarily used as sequestrants and water softeners .
Properties
Key on ui mechanism of action |
ARSONIC ACID HERBICIDES ARE NOT GROWTH REGULATORS IN SENSE OF PLANT HORMONES. THEY ... ACT THROUGH ENZYME SYSTEMS TO INHIBIT GROWTH. THEY KILL ... SLOWLY; FIRST SYMPTOMS ARE ... CHLOROSIS, CESSATION OF GROWTH, & GRADUAL YELLOWING, THEN BROWNING FOLLOWED BY DEHYDRATION & DEATH. /ORGANOARSENICAL HERBICIDES/ |
---|---|
CAS No. |
7758-16-9 |
Molecular Formula |
H4NaO7P2 |
Molecular Weight |
200.96 g/mol |
IUPAC Name |
disodium;[hydroxy(oxido)phosphoryl] hydrogen phosphate |
InChI |
InChI=1S/Na.H4O7P2/c;1-8(2,3)7-9(4,5)6/h;(H2,1,2,3)(H2,4,5,6) |
InChI Key |
MHXGNUVRVJWHJK-UHFFFAOYSA-N |
SMILES |
OP(=O)([O-])OP(=O)(O)[O-].[Na+].[Na+] |
Canonical SMILES |
OP(=O)(O)OP(=O)(O)O.[Na] |
boiling_point |
Decomposes (NIOSH, 2024) Decomposes |
Color/Form |
White crystalline powder |
density |
Density: 1.86 /Hexahydrate/ |
melting_point |
1810 °F (NIOSH, 2024) 988 °C 1810 °F |
Key on ui other cas no. |
68915-31-1 7758-16-9 7782-95-8 |
physical_description |
Liquid; Other Solid; Pellets or Large Crystals; Dry Powder White powder or grains White solid; [Merck Index] White odorless powder; [MSDSonline] |
Pictograms |
Corrosive; Irritant |
Related CAS |
10042-91-8 |
solubility |
Soluble in water Sol in wate |
vapor_pressure |
0 mmHg (approx) (NIOSH, 2024) 0 mmHg (approx) |
Origin of Product |
United States |
Retrosynthesis Analysis
AI-Powered Synthesis Planning: Our tool employs the Template_relevance Pistachio, Template_relevance Bkms_metabolic, Template_relevance Pistachio_ringbreaker, Template_relevance Reaxys, Template_relevance Reaxys_biocatalysis model, leveraging a vast database of chemical reactions to predict feasible synthetic routes.
One-Step Synthesis Focus: Specifically designed for one-step synthesis, it provides concise and direct routes for your target compounds, streamlining the synthesis process.
Accurate Predictions: Utilizing the extensive PISTACHIO, BKMS_METABOLIC, PISTACHIO_RINGBREAKER, REAXYS, REAXYS_BIOCATALYSIS database, our tool offers high-accuracy predictions, reflecting the latest in chemical research and data.
Strategy Settings
Precursor scoring | Relevance Heuristic |
---|---|
Min. plausibility | 0.01 |
Model | Template_relevance |
Template Set | Pistachio/Bkms_metabolic/Pistachio_ringbreaker/Reaxys/Reaxys_biocatalysis |
Top-N result to add to graph | 6 |
Feasible Synthetic Routes
Q1: How does SAPP impact the texture of meat products?
A1: SAPP can improve the texture of meat products by enhancing binding ability, increasing firmness and springiness, and reducing oiliness. This was observed in studies on frankfurters [] and restructured pork jerky [].
Q2: Can SAPP be used to reduce the need for sodium nitrite in meat products?
A3: Research suggests that while SAPP can contribute to some desirable qualities in reduced-sodium nitrite frankfurters, it cannot fully replace nitrite's role in color development and flavor [].
Q3: Does SAPP impact the shelf life of meat products?
A4: Studies indicate that SAPP, especially in combination with other preservatives like potassium sorbate, can effectively inhibit bacterial growth in meat products, thus extending their shelf life [, ].
Q4: How does SAPP affect the stability of strawberry puree during processing?
A5: SAPP, in combination with ascorbic acid, helps retain the anthocyanin content and red color intensity of strawberry puree during processing [].
Q5: Can SAPP be used to prevent darkening in potatoes?
A6: Yes, SAPP has been used as an anti-browning agent in the potato industry. It helps reduce after-cooking darkening in potatoes, though its effectiveness might be lower compared to other agents like citric acid [, , ].
Q6: Does SAPP affect the quality of frozen fish fillets?
A7: Studies on Nile tilapia fillets showed that SAPP, specifically sodium tripolyphosphate (STPP), improved weight gain, cooking yield, and reduced drip and cooking loss. It also slightly enhanced sensory acceptability [].
Q7: How does SAPP function as a leavening agent in baked goods?
A8: While SAPP itself doesn't release CO2, it reacts with sodium bicarbonate in baking powder. This reaction generates CO2, contributing to the aeration and volume of baked goods like biscuits and cakes [, , ].
Q8: How does SAPP interact with other ingredients in baked goods?
A9: Thermal analysis studies showed that SAPP interacts with other ingredients during baking. It can lower the dough rise temperature and its effect on the dough's thermal behavior is influenced by the presence of fats and sugars [].
Q9: What is the molecular formula and weight of SAPP?
A9: The molecular formula of SAPP is Na2H2P2O7, and its molecular weight is 221.94 g/mol.
Q10: How does the type of phosphate salt affect its functionality in meat products?
A11: Different phosphate salts exhibit varying impacts on meat product qualities. For example, STPP showed better water holding capacity and yield in turkey frankfurters compared to SAPP and SHMP [, ].
Q11: Do different phosphate salts have different antimicrobial effects?
A12: Yes, research on bratwurst indicated that SAPP had a greater inhibitory effect on bacterial growth during temperature abuse compared to other phosphates like STPP and TSPP [, ].
Q12: How does the pH of the food matrix affect SAPP's functionality?
A13: The pH of the food matrix significantly influences SAPP's effectiveness. For instance, in meat batters, the antimicrobial activity of SAPP was enhanced at lower pH levels [, ].
Q13: Can SAPP be encapsulated to improve its application in food products?
A14: Yes, research has shown that SAPP can be encapsulated within food-grade carbohydrates, such as β-cyclodextrin, to improve the stability and controlled release of the compound in meat products [].
Q14: Are there any concerns regarding the use of phosphates in food?
A16: Excessive phosphate intake has been linked to health concerns like cardiovascular disease and bone health issues. Therefore, it is essential to use phosphates judiciously in food processing [].
Q15: Are there any alternatives to SAPP in food applications?
A17: Yes, alternative food-grade phosphates like STPP, TSPP, and SHMP are often used. Additionally, natural acids like citric acid and lactic acid can be considered as substitutes for specific applications [, , , , ].
Q16: What analytical techniques are used to study the interaction of SAPP with food components?
A18: Various techniques are employed, including spectrophotometry to assess color changes [, , ], texture analysis for evaluating textural modifications [, , ], and microbiological assays for determining antimicrobial efficacy [, , ].
Disclaimer and Information on In-Vitro Research Products
Please be aware that all articles and product information presented on BenchChem are intended solely for informational purposes. The products available for purchase on BenchChem are specifically designed for in-vitro studies, which are conducted outside of living organisms. In-vitro studies, derived from the Latin term "in glass," involve experiments performed in controlled laboratory settings using cells or tissues. It is important to note that these products are not categorized as medicines or drugs, and they have not received approval from the FDA for the prevention, treatment, or cure of any medical condition, ailment, or disease. We must emphasize that any form of bodily introduction of these products into humans or animals is strictly prohibited by law. It is essential to adhere to these guidelines to ensure compliance with legal and ethical standards in research and experimentation.