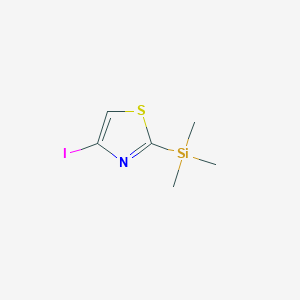
4-Iodo-2-(trimethylsilyl)-1,3-thiazole
- Click on QUICK INQUIRY to receive a quote from our team of experts.
- With the quality product at a COMPETITIVE price, you can focus more on your research.
Overview
Description
4-Iodo-2-(trimethylsilyl)-1,3-thiazole is a halogenated, silicon-containing derivative of the 1,3-thiazole scaffold. The 1,3-thiazole ring is a five-membered heterocycle with sulfur and nitrogen atoms at positions 1 and 3, respectively. This compound features an iodine atom at the 4-position and a trimethylsilyl (TMS) group at the 2-position.
1,3-Thiazoles are pharmacologically significant, with derivatives exhibiting anti-inflammatory, antimicrobial, and antitumoral activities .
Preparation Methods
Synthetic Routes and Reaction Conditions: The synthesis of 4-Iodo-2-(trimethylsilyl)-1,3-thiazole typically involves the iodination of a thiazole precursor followed by the introduction of the trimethylsilyl group. One common method involves the reaction of 2-(trimethylsilyl)-1,3-thiazole with iodine in the presence of a suitable oxidizing agent. The reaction is usually carried out in an inert atmosphere to prevent unwanted side reactions.
Industrial Production Methods: While specific industrial production methods for this compound are not well-documented, the general approach would involve scaling up the laboratory synthesis methods. This would include optimizing reaction conditions to maximize yield and purity, as well as implementing safety measures to handle the reactive iodine and trimethylsilyl reagents.
Chemical Reactions Analysis
Types of Reactions: 4-Iodo-2-(trimethylsilyl)-1,3-thiazole can undergo various chemical reactions, including:
Substitution Reactions: The iodine atom can be replaced by other nucleophiles, such as amines or thiols, under suitable conditions.
Oxidation and Reduction: The thiazole ring can participate in oxidation and reduction reactions, altering the electronic properties of the compound.
Coupling Reactions: The trimethylsilyl group can be used to facilitate cross-coupling reactions, such as Suzuki or Stille couplings, to form new carbon-carbon bonds.
Common Reagents and Conditions:
Substitution Reactions: Typically involve nucleophiles like amines or thiols in the presence of a base.
Oxidation: Can be carried out using oxidizing agents like hydrogen peroxide or m-chloroperbenzoic acid.
Reduction: Often involves reducing agents such as lithium aluminum hydride or sodium borohydride.
Coupling Reactions: Utilize palladium catalysts and appropriate ligands under inert conditions.
Major Products: The products of these reactions vary depending on the specific reagents and conditions used. For example, substitution reactions can yield a variety of functionalized thiazoles, while coupling reactions can produce complex organic molecules with extended conjugation.
Scientific Research Applications
4-Iodo-2-(trimethylsilyl)-1,3-thiazole has several applications in scientific research:
Organic Synthesis: Used as a building block for the synthesis of more complex molecules, particularly in the development of pharmaceuticals and agrochemicals.
Materials Science:
Medicinal Chemistry: Investigated for its potential biological activity and as a precursor for the synthesis of bioactive compounds.
Chemical Biology: Used in the study of enzyme mechanisms and as a probe for investigating biological pathways.
Mechanism of Action
The mechanism of action of 4-Iodo-2-(trimethylsilyl)-1,3-thiazole depends on its specific application. In organic synthesis, it acts as a versatile intermediate that can undergo various transformations. In biological systems, its mechanism would be determined by the specific molecular targets it interacts with, such as enzymes or receptors. The trimethylsilyl group can enhance the compound’s stability and facilitate its transport across cell membranes, while the iodine atom can participate in halogen bonding interactions.
Comparison with Similar Compounds
The structural and functional properties of 4-Iodo-2-(trimethylsilyl)-1,3-thiazole are compared below with analogous 1,3-thiazole derivatives (Table 1) and discussed in detail.
Table 1: Structural and Functional Comparison of 1,3-Thiazole Derivatives
Halogenated Derivatives
- 4-Bromo-2-(trimethylsilyl)-1,3-thiazole : This bromine-substituted analog shares the TMS group but exhibits lower molecular weight and reduced steric hindrance compared to the iodine derivative. Bromine’s smaller atomic radius and weaker electron-withdrawing effects may result in divergent reactivity in cross-coupling reactions .
- Iodine vs.
Alkyl- and Alkyne-Substituted Derivatives
- 4-Ethyl-2-ethynyl-1,3-thiazole : The ethynyl group enables click chemistry applications, while the ethyl substituent increases lipophilicity. However, this compound shows only moderate antimicrobial activity (MIC: 25–200 µg/mL), suggesting that bulky substituents (e.g., TMS) may enhance target engagement .
- 2-Methyl-4-isopropyl-1,3-thiazole: Alkyl substituents confer volatility, making this compound useful in flavor/fragrance industries.
Hybrid Scaffolds
- Thiazole-1,3,4-oxadiazole hybrids : These hybrids (e.g., compound 9c ) demonstrate potent anticancer activity (IC50: 12 µM against HT-29 cells) due to synergistic effects from the oxadiazole moiety, which enhances π-π stacking and hydrogen bonding with biological targets .
- Thiosemicarbazone-thiazoles: Pyridyl-thiazole derivatives (e.g., from ) regulate immune factors (e.g., IL-10, TNF-α) and induce apoptosis, highlighting the importance of nitrogen-rich substituents for immunomodulatory activity .
Electronic and Steric Effects
- Its electron-donating nature may also stabilize the thiazole ring .
- Substituent Position : Derivatives with substituents at the 4-position (e.g., iodine, bromine) show enhanced bioactivity compared to 2-position analogs, as seen in antimicrobial studies where 4-hydroxyphenyl-substituted thiazoles outperformed isomers .
Research Findings and Implications
Synthetic Utility : The iodine and TMS groups in 4-Iodo-2-TMS-thiazole make it a versatile intermediate for synthesizing complex heterocycles via cross-coupling or silylation reactions .
Further in vitro studies are needed to validate these hypotheses .
Structure-Activity Relationship (SAR) : Bulky, electronegative substituents at the 4-position enhance target binding, while hybrid scaffolds improve pharmacokinetic properties .
Biological Activity
4-Iodo-2-(trimethylsilyl)-1,3-thiazole is a heterocyclic compound with significant biological activity due to its unique structural features. This compound contains a thiazole ring, which is characterized by the presence of sulfur and nitrogen atoms, along with an iodine atom at the 4-position and a trimethylsilyl group at the 2-position. The molecular formula for this compound is C₅H₈I₁N₁S₁, and it has a CAS number of 533887-52-4. The thiazole ring structure contributes to its diverse pharmacological properties, making it a subject of interest in various chemical and biological studies.
Antimicrobial Properties
Compounds containing thiazole rings, including this compound, are known for their antimicrobial activities. Studies indicate that derivatives of thiazoles exhibit significant biological activities due to their ability to interact with various biological targets such as enzymes and receptors. For instance, thiazole derivatives have been shown to possess antibacterial and antifungal properties:
- Antibacterial Activity : Research has demonstrated that certain thiazole derivatives can inhibit the growth of both Gram-positive and Gram-negative bacteria. The minimum inhibitory concentration (MIC) values for some derivatives range from 0.06 to 1.88 mg/mL against various bacterial strains .
- Antifungal Activity : Thiazole compounds have also shown efficacy against fungal strains, with MIC values ranging from 6.25 to 12.5 µg/mL against species like Aspergillus fumigatus and Trichophyton mentagrophytes .
Anticancer Activity
The potential anticancer properties of thiazole derivatives are also noteworthy. Some studies have indicated that these compounds can induce apoptosis in cancer cells through various mechanisms, such as the inhibition of specific signaling pathways or the modulation of gene expression related to cell survival.
Interaction with Biological Targets
The presence of both iodine and a trimethylsilyl group in this compound enhances its reactivity towards biological targets. The trimethylsilyl group can influence the electronic properties of the thiazole ring, affecting its interaction with enzymes and receptors involved in disease processes.
Comparative Analysis
To understand the uniqueness of this compound in comparison to similar compounds, the following table summarizes key features:
Compound Name | Structure Features | Unique Aspects |
---|---|---|
4-Iodo-1,3-thiazole | Iodine at position 4; no trimethylsilyl group | Lacks the trimethylsilyl group |
2-(Trimethylsilyl)-1,3-thiazole | Trimethylsilyl group at position 2; no iodine | Lacks halogen substitution |
4-Bromo-2-(trimethylsilyl)-1,3-thiazole | Bromine instead of iodine; trimethylsilyl present | Different halogen affects reactivity |
5-Methylthiazole | Methyl group instead of iodine; no silylation | Different substituent pattern affects properties |
The distinctive reactivity and potential applications of this compound can be attributed to its unique combination of substituents.
Study on Antimicrobial Activity
A study evaluated a series of thiazole derivatives for their antimicrobial activity against various pathogens. Among them, compounds with specific substituents showed enhanced activity against resistant strains such as methicillin-resistant Staphylococcus aureus (MRSA). The most active derivatives exhibited MIC values significantly lower than traditional antibiotics like ampicillin .
Neuropharmacological Applications
Another research highlighted the use of iodo-thiazoles in neuropharmacological studies. For instance, [11C]SP203, derived from thiazole precursors including iodine substitutions, was used in PET imaging to study mGluR5 receptor occupancy in primate models. This application underscores the potential utility of thiazole derivatives in drug development and neurological research .
Q & A
Basic Research Questions
Q. What are the optimal synthetic routes for 4-Iodo-2-(trimethylsilyl)-1,3-thiazole, and how can reaction conditions be systematically optimized?
- Methodological Answer : The synthesis typically involves coupling reactions between iodinated precursors and trimethylsilyl-containing intermediates. For example, Sonogashira or Stille couplings under palladium catalysis (e.g., Pd(PPh₃)₄) in anhydrous THF or DMF at 60–80°C . Optimization can employ statistical design of experiments (DoE), such as factorial designs, to evaluate variables like temperature, solvent polarity, and catalyst loading. For instance, highlights DoE's role in minimizing experimental runs while maximizing yield . Reaction progress should be monitored via TLC and NMR to confirm intermediate formation .
Q. How should researchers characterize the structure and purity of this compound?
- Methodological Answer : Use a combination of spectroscopic techniques:
- ¹H/¹³C NMR : Confirm the presence of the trimethylsilyl group (δ ~0.3 ppm for Si(CH₃)₃) and thiazole ring protons (δ 7.5–8.5 ppm) .
- IR Spectroscopy : Identify C-I (500–600 cm⁻¹) and Si-C (1250–1300 cm⁻¹) stretches .
- Elemental Analysis : Compare experimental C, H, N, and S percentages with theoretical values to confirm purity (±0.3% tolerance) .
Q. What solvent systems and storage conditions ensure the stability of this compound?
- Methodological Answer : The compound is moisture-sensitive due to the trimethylsilyl group. Store under inert atmosphere (Ar/N₂) in anhydrous solvents like dichloromethane or THF at –20°C. Avoid protic solvents (e.g., MeOH, H₂O) to prevent hydrolysis. Stability tests via ¹H NMR over 72 hours can detect degradation .
Q. How can researchers troubleshoot low yields in the iodination step of thiazole derivatives?
- Methodological Answer : Low iodination efficiency may arise from incomplete activation of the thiazole ring. Pre-functionalize the thiazole with electron-withdrawing groups (e.g., nitro) to enhance electrophilic substitution. Alternatively, use N-iodosuccinimide (NIS) in acetic acid at 50°C, as iodine incorporation is favored in polar aprotic solvents . Monitor iodine consumption via GC-MS or iodometric titration .
Q. What analytical techniques are critical for confirming regioselectivity in thiazole functionalization?
- Methodological Answer : Regioselectivity can be validated via:
- NOESY NMR : Detect spatial proximity between substituents (e.g., iodine and trimethylsilyl groups) .
- X-ray Crystallography : Resolve crystal structures to confirm substitution patterns .
- HPLC-MS : Compare retention times and fragmentation patterns with synthetic standards .
Advanced Research Questions
Q. How can computational chemistry predict the reactivity of this compound in cross-coupling reactions?
- Methodological Answer : Density functional theory (DFT) calculations (e.g., B3LYP/6-31G*) model transition states for coupling reactions. For example, calculate activation energies for Suzuki-Miyaura reactions with aryl boronic acids to identify steric/electronic effects of the trimethylsilyl group. ICReDD’s approach integrates quantum chemical reaction path searches with experimental validation to optimize conditions .
Q. What strategies resolve contradictions in spectroscopic data for derivatives of this compound?
- Methodological Answer : Conflicting NMR signals may arise from dynamic processes (e.g., ring puckering). Use variable-temperature NMR to freeze conformational changes. For ambiguous IR peaks, employ isotopic labeling (e.g., deuterated solvents) or 2D-COSY to decouple overlapping signals . Compare experimental data with simulated spectra from Gaussian or ADF software .
Q. How does the trimethylsilyl group influence the electronic properties of the thiazole ring in catalytic applications?
- Methodological Answer : The trimethylsilyl group acts as a σ-donor, increasing electron density on the thiazole ring. Cyclic voltammetry (CV) can measure oxidation potentials (e.g., E₁/2 shifts by –0.2 V vs. non-silylated analogs). DFT-based NBO analysis quantifies charge distribution, revealing enhanced nucleophilicity at the C-5 position .
Q. What experimental designs minimize byproducts during functionalization of this compound?
- Methodological Answer : Use DoE to screen factors like stoichiometry, catalyst type (e.g., Pd vs. Cu), and solvent polarity. For example, a central composite design (CCD) identified that CuI/1,10-phenanthroline in DMF at 100°C maximizes Sonogashira coupling yields (>85%) while minimizing homo-coupling byproducts (<5%) . Post-reaction purification via column chromatography (silica gel, hexane/EtOAc) isolates desired products .
Q. How can researchers leverage this compound in multi-component reactions (MCRs) for heterocyclic diversity?
- Methodological Answer :
The iodine substituent enables sequential cross-coupling and cycloaddition. For example: - Step 1 : Suzuki coupling with boronic acids to install aryl groups.
- Step 2 : Cu-catalyzed azide-alkyne cycloaddition (CuAAC) to form triazole rings .
Reaction progress is tracked via in-situ FTIR or LC-MS. Optimize MCRs using microfluidic reactors for precise control of mixing and residence time .
Properties
Molecular Formula |
C6H10INSSi |
---|---|
Molecular Weight |
283.21 g/mol |
IUPAC Name |
(4-iodo-1,3-thiazol-2-yl)-trimethylsilane |
InChI |
InChI=1S/C6H10INSSi/c1-10(2,3)6-8-5(7)4-9-6/h4H,1-3H3 |
InChI Key |
VTLSOUSMUHMXQP-UHFFFAOYSA-N |
Canonical SMILES |
C[Si](C)(C)C1=NC(=CS1)I |
Origin of Product |
United States |
Disclaimer and Information on In-Vitro Research Products
Please be aware that all articles and product information presented on BenchChem are intended solely for informational purposes. The products available for purchase on BenchChem are specifically designed for in-vitro studies, which are conducted outside of living organisms. In-vitro studies, derived from the Latin term "in glass," involve experiments performed in controlled laboratory settings using cells or tissues. It is important to note that these products are not categorized as medicines or drugs, and they have not received approval from the FDA for the prevention, treatment, or cure of any medical condition, ailment, or disease. We must emphasize that any form of bodily introduction of these products into humans or animals is strictly prohibited by law. It is essential to adhere to these guidelines to ensure compliance with legal and ethical standards in research and experimentation.