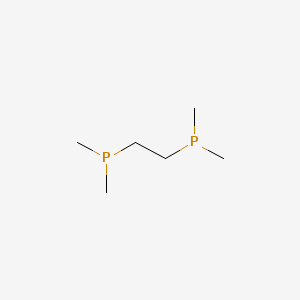
1,2-Bis(dimethylphosphino)ethane
Overview
Description
1,2-Bis(dimethylphosphino)ethane (dmpe) is a diphosphine ligand used in coordination chemistry. It is a colorless, air-sensitive liquid that is soluble in organic solvents .
Synthesis Analysis
The compound is synthesized by the reaction of methylmagnesium iodide with 1,2-bis(dichlorophosphino)ethane . Alternatively, it can be generated by alkylation of sodium dimethylphosphide .Molecular Structure Analysis
The molecular formula of 1,2-Bis(dimethylphosphino)ethane is C6H16P2. It has an average mass of 150.139 Da and a monoisotopic mass of 150.072723 Da . The structure features two dimethylphosphine groups (PMe2) connected by an ethane chain (CH2CH2).Chemical Reactions Analysis
1,2-Bis(dimethylphosphino)ethane reacts with ZrCl2 to afford ZrCl4 (dmpe)2 complexes . The reduction of dppe by lithium to give PhHP(CH2)2PHPh has also been reported .Physical And Chemical Properties Analysis
The compound has a boiling point of 181.1±23.0 °C at 760 mmHg and a density of 0.9 g/mL at 25 °C . It has a refractive index of n20/D 1.507 (lit.) .Scientific Research Applications
Catalysis in Organic Synthesis
dmpe: acts as a chelating ligand in transition metal complexes that are used as catalysts in organic synthesis. Its ability to stabilize metal centers enhances the efficiency of reactions such as:
- Buchwald-Hartwig Cross Coupling Reaction : Used to form carbon-nitrogen bonds .
- Heck Reaction : Facilitates the formation of carbon-carbon bonds between alkenes and aryl or vinyl halides .
- Suzuki-Miyaura Coupling : A pivotal reaction for forming biaryl compounds, which are essential in pharmaceuticals and organic materials .
Homogeneous Catalysis
dmpe: is employed in homogeneous catalysis where it forms complexes with metals like iridium. These complexes can activate small molecules such as methane, enabling reactions like:
- Selective Monoborylation of Methane : The use of dmpe with iridium catalysts lowers the activation barrier, allowing for the borylation of methane at lower temperatures .
Coordination Chemistry
In coordination chemistry, dmpe is used to synthesize various metal complexes due to its strong electron-donating properties and the ability to form stable five-membered rings with metal centers. Examples include:
- Transition Metal Complexes : Formation of complexes with metals like nickel, palladium, and zirconium which have applications in catalysis and material science .
Material Science
dmpe: -based metal complexes have potential applications in material science, such as:
- Molecular Electronics : Metal complexes with dmpe can exhibit properties useful in the development of molecular electronic devices .
Pharmaceutical Research
Metal complexes with dmpe ligands are explored for their medicinal properties, including:
- Anticancer Agents : Certain metal-dmpe complexes are studied for their role as potential anticancer drugs .
Environmental Chemistry
dmpe: complexes are used in environmental chemistry for:
- Carbon Capture : Research into the use of dmpe-metal complexes for capturing and converting carbon dioxide is ongoing .
Polymerization Processes
In polymer science, dmpe is used to create catalysts for:
- Olefin Polymerization : Catalysts containing dmpe ligands can be used to polymerize olefins, which are the building blocks for various plastics .
Nuclear Magnetic Resonance (NMR) Spectroscopy
dmpe: can be used in NMR spectroscopy as:
Mechanism of Action
Target of Action
1,2-Bis(dimethylphosphino)ethane (dmpe) is a diphosphine ligand in coordination chemistry . It is used as a compact, strongly basic spectator ligand . The primary targets of dmpe are metal ions, with which it forms complexes .
Mode of Action
Dmpe interacts with its targets by donating electron pairs to the metal ions, forming metal-ligand bonds . For example, it reacts with ZrCl2 to afford ZrCl4(dmpe)2 complexes . The formation of these complexes can alter the chemical properties of the metal ions, enabling them to participate in various chemical reactions .
Biochemical Pathways
The specific biochemical pathways affected by dmpe depend on the nature of the metal ion it is complexed with. As a ligand, dmpe can influence the reactivity of the metal center and stabilize certain oxidation states, thereby affecting the course of the chemical reactions .
Pharmacokinetics
Its elimination would likely occur through metabolic processes, although the specifics would depend on the nature of the metal complex formed .
Result of Action
The molecular and cellular effects of dmpe’s action are largely dependent on the metal ion it is complexed with. In general, the formation of metal-ligand complexes can enable or enhance various chemical reactions, potentially leading to changes in molecular structures or cellular processes .
Safety and Hazards
Future Directions
properties
IUPAC Name |
2-dimethylphosphanylethyl(dimethyl)phosphane | |
---|---|---|
Source | PubChem | |
URL | https://pubchem.ncbi.nlm.nih.gov | |
Description | Data deposited in or computed by PubChem | |
InChI |
InChI=1S/C6H16P2/c1-7(2)5-6-8(3)4/h5-6H2,1-4H3 | |
Source | PubChem | |
URL | https://pubchem.ncbi.nlm.nih.gov | |
Description | Data deposited in or computed by PubChem | |
InChI Key |
ZKWQSBFSGZJNFP-UHFFFAOYSA-N | |
Source | PubChem | |
URL | https://pubchem.ncbi.nlm.nih.gov | |
Description | Data deposited in or computed by PubChem | |
Canonical SMILES |
CP(C)CCP(C)C | |
Source | PubChem | |
URL | https://pubchem.ncbi.nlm.nih.gov | |
Description | Data deposited in or computed by PubChem | |
Molecular Formula |
C6H16P2 | |
Source | PubChem | |
URL | https://pubchem.ncbi.nlm.nih.gov | |
Description | Data deposited in or computed by PubChem | |
DSSTOX Substance ID |
DTXSID70178649 | |
Record name | 1,2-Bis(dimethylphosphino)ethane | |
Source | EPA DSSTox | |
URL | https://comptox.epa.gov/dashboard/DTXSID70178649 | |
Description | DSSTox provides a high quality public chemistry resource for supporting improved predictive toxicology. | |
Molecular Weight |
150.14 g/mol | |
Source | PubChem | |
URL | https://pubchem.ncbi.nlm.nih.gov | |
Description | Data deposited in or computed by PubChem | |
CAS RN |
23936-60-9 | |
Record name | 1,2-Bis(dimethylphosphino)ethane | |
Source | CAS Common Chemistry | |
URL | https://commonchemistry.cas.org/detail?cas_rn=23936-60-9 | |
Description | CAS Common Chemistry is an open community resource for accessing chemical information. Nearly 500,000 chemical substances from CAS REGISTRY cover areas of community interest, including common and frequently regulated chemicals, and those relevant to high school and undergraduate chemistry classes. This chemical information, curated by our expert scientists, is provided in alignment with our mission as a division of the American Chemical Society. | |
Explanation | The data from CAS Common Chemistry is provided under a CC-BY-NC 4.0 license, unless otherwise stated. | |
Record name | 1,2-Bis(dimethylphosphino)ethane | |
Source | ChemIDplus | |
URL | https://pubchem.ncbi.nlm.nih.gov/substance/?source=chemidplus&sourceid=0023936609 | |
Description | ChemIDplus is a free, web search system that provides access to the structure and nomenclature authority files used for the identification of chemical substances cited in National Library of Medicine (NLM) databases, including the TOXNET system. | |
Record name | 1,2-Bis(dimethylphosphino)ethane | |
Source | EPA DSSTox | |
URL | https://comptox.epa.gov/dashboard/DTXSID70178649 | |
Description | DSSTox provides a high quality public chemistry resource for supporting improved predictive toxicology. | |
Record name | 1,2-Bis(dimethylphosphino)ethane | |
Source | European Chemicals Agency (ECHA) | |
URL | https://echa.europa.eu/information-on-chemicals | |
Description | The European Chemicals Agency (ECHA) is an agency of the European Union which is the driving force among regulatory authorities in implementing the EU's groundbreaking chemicals legislation for the benefit of human health and the environment as well as for innovation and competitiveness. | |
Explanation | Use of the information, documents and data from the ECHA website is subject to the terms and conditions of this Legal Notice, and subject to other binding limitations provided for under applicable law, the information, documents and data made available on the ECHA website may be reproduced, distributed and/or used, totally or in part, for non-commercial purposes provided that ECHA is acknowledged as the source: "Source: European Chemicals Agency, http://echa.europa.eu/". Such acknowledgement must be included in each copy of the material. ECHA permits and encourages organisations and individuals to create links to the ECHA website under the following cumulative conditions: Links can only be made to webpages that provide a link to the Legal Notice page. | |
Record name | 1,2-BIS(DIMETHYLPHOSPHINO)ETHANE | |
Source | FDA Global Substance Registration System (GSRS) | |
URL | https://gsrs.ncats.nih.gov/ginas/app/beta/substances/9P3522CZ8T | |
Description | The FDA Global Substance Registration System (GSRS) enables the efficient and accurate exchange of information on what substances are in regulated products. Instead of relying on names, which vary across regulatory domains, countries, and regions, the GSRS knowledge base makes it possible for substances to be defined by standardized, scientific descriptions. | |
Explanation | Unless otherwise noted, the contents of the FDA website (www.fda.gov), both text and graphics, are not copyrighted. They are in the public domain and may be republished, reprinted and otherwise used freely by anyone without the need to obtain permission from FDA. Credit to the U.S. Food and Drug Administration as the source is appreciated but not required. | |
Retrosynthesis Analysis
AI-Powered Synthesis Planning: Our tool employs the Template_relevance Pistachio, Template_relevance Bkms_metabolic, Template_relevance Pistachio_ringbreaker, Template_relevance Reaxys, Template_relevance Reaxys_biocatalysis model, leveraging a vast database of chemical reactions to predict feasible synthetic routes.
One-Step Synthesis Focus: Specifically designed for one-step synthesis, it provides concise and direct routes for your target compounds, streamlining the synthesis process.
Accurate Predictions: Utilizing the extensive PISTACHIO, BKMS_METABOLIC, PISTACHIO_RINGBREAKER, REAXYS, REAXYS_BIOCATALYSIS database, our tool offers high-accuracy predictions, reflecting the latest in chemical research and data.
Strategy Settings
Precursor scoring | Relevance Heuristic |
---|---|
Min. plausibility | 0.01 |
Model | Template_relevance |
Template Set | Pistachio/Bkms_metabolic/Pistachio_ringbreaker/Reaxys/Reaxys_biocatalysis |
Top-N result to add to graph | 6 |
Feasible Synthetic Routes
Disclaimer and Information on In-Vitro Research Products
Please be aware that all articles and product information presented on BenchChem are intended solely for informational purposes. The products available for purchase on BenchChem are specifically designed for in-vitro studies, which are conducted outside of living organisms. In-vitro studies, derived from the Latin term "in glass," involve experiments performed in controlled laboratory settings using cells or tissues. It is important to note that these products are not categorized as medicines or drugs, and they have not received approval from the FDA for the prevention, treatment, or cure of any medical condition, ailment, or disease. We must emphasize that any form of bodily introduction of these products into humans or animals is strictly prohibited by law. It is essential to adhere to these guidelines to ensure compliance with legal and ethical standards in research and experimentation.