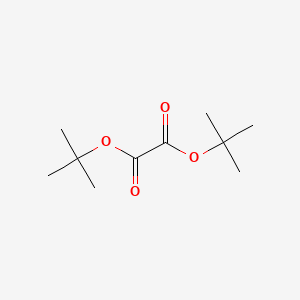
Di-tert-butyl oxalate
Overview
Description
Di-tert-butyl oxalate is an organic compound with the molecular formula (CH₃)₃COCOCOOC(CH₃)₃. It is an ester derived from oxalic acid and tert-butyl alcohol. This compound is known for its use as a reagent in organic synthesis and has applications in various chemical reactions.
Preparation Methods
Synthetic Routes and Reaction Conditions: Di-tert-butyl oxalate can be synthesized through the esterification of oxalic acid with tert-butyl alcohol. The reaction typically involves the use of a dehydrating agent such as sulfuric acid or a catalyst like p-toluenesulfonic acid to facilitate the esterification process. The reaction is carried out under reflux conditions to ensure complete conversion of the reactants to the desired ester.
Industrial Production Methods: In an industrial setting, the production of this compound may involve continuous flow processes to enhance efficiency and yield. The use of automated systems and optimized reaction conditions can help in scaling up the production while maintaining the purity and quality of the compound.
Chemical Reactions Analysis
Types of Reactions: Di-tert-butyl oxalate undergoes various chemical reactions, including:
Hydrolysis: In the presence of water and an acid or base catalyst, this compound can hydrolyze to form oxalic acid and tert-butyl alcohol.
Reduction: It can be reduced to form di-tert-butyl glycolate using reducing agents like lithium aluminum hydride.
Substitution: The ester group in this compound can participate in nucleophilic substitution reactions, leading to the formation of different derivatives.
Common Reagents and Conditions:
Hydrolysis: Acidic or basic conditions with water.
Reduction: Lithium aluminum hydride in anhydrous ether.
Substitution: Nucleophiles such as amines or alcohols under mild conditions.
Major Products Formed:
Hydrolysis: Oxalic acid and tert-butyl alcohol.
Reduction: Di-tert-butyl glycolate.
Substitution: Various substituted oxalates depending on the nucleophile used.
Scientific Research Applications
Di-tert-butyl oxalate has several applications in scientific research:
Chemistry: It is used as a reagent in organic synthesis for the preparation of various oxalate derivatives.
Biology: It can be used in the synthesis of biologically active compounds and as a protecting group for carboxylic acids.
Medicine: Research into potential pharmaceutical applications, including drug synthesis and development.
Industry: Utilized in the production of specialty chemicals and materials.
Mechanism of Action
The mechanism of action of di-tert-butyl oxalate involves its reactivity as an ester. In hydrolysis reactions, the ester bond is cleaved by nucleophilic attack from water, leading to the formation of oxalic acid and tert-butyl alcohol. In reduction reactions, the ester group is reduced to an alcohol by the transfer of hydride ions from the reducing agent.
Comparison with Similar Compounds
Diethyl oxalate: Another ester of oxalic acid, but with ethyl groups instead of tert-butyl groups.
Dibenzyl oxalate: An ester of oxalic acid with benzyl groups.
Dimethyl oxalate: An ester of oxalic acid with methyl groups.
Comparison: Di-tert-butyl oxalate is unique due to the bulky tert-butyl groups, which provide steric hindrance and influence its reactivity compared to other oxalate esters. This steric effect can affect the compound’s behavior in chemical reactions, making it a valuable reagent in specific synthetic applications where such properties are desired.
Properties
IUPAC Name |
ditert-butyl oxalate | |
---|---|---|
Source | PubChem | |
URL | https://pubchem.ncbi.nlm.nih.gov | |
Description | Data deposited in or computed by PubChem | |
InChI |
InChI=1S/C10H18O4/c1-9(2,3)13-7(11)8(12)14-10(4,5)6/h1-6H3 | |
Source | PubChem | |
URL | https://pubchem.ncbi.nlm.nih.gov | |
Description | Data deposited in or computed by PubChem | |
InChI Key |
CIYGMWIAXRMHQS-UHFFFAOYSA-N | |
Source | PubChem | |
URL | https://pubchem.ncbi.nlm.nih.gov | |
Description | Data deposited in or computed by PubChem | |
Canonical SMILES |
CC(C)(C)OC(=O)C(=O)OC(C)(C)C | |
Source | PubChem | |
URL | https://pubchem.ncbi.nlm.nih.gov | |
Description | Data deposited in or computed by PubChem | |
Molecular Formula |
C10H18O4 | |
Source | PubChem | |
URL | https://pubchem.ncbi.nlm.nih.gov | |
Description | Data deposited in or computed by PubChem | |
DSSTOX Substance ID |
DTXSID20219157 | |
Record name | Di-tert-butyl oxalate | |
Source | EPA DSSTox | |
URL | https://comptox.epa.gov/dashboard/DTXSID20219157 | |
Description | DSSTox provides a high quality public chemistry resource for supporting improved predictive toxicology. | |
Molecular Weight |
202.25 g/mol | |
Source | PubChem | |
URL | https://pubchem.ncbi.nlm.nih.gov | |
Description | Data deposited in or computed by PubChem | |
CAS No. |
691-64-5 | |
Record name | 1,2-Bis(1,1-dimethylethyl) ethanedioate | |
Source | CAS Common Chemistry | |
URL | https://commonchemistry.cas.org/detail?cas_rn=691-64-5 | |
Description | CAS Common Chemistry is an open community resource for accessing chemical information. Nearly 500,000 chemical substances from CAS REGISTRY cover areas of community interest, including common and frequently regulated chemicals, and those relevant to high school and undergraduate chemistry classes. This chemical information, curated by our expert scientists, is provided in alignment with our mission as a division of the American Chemical Society. | |
Explanation | The data from CAS Common Chemistry is provided under a CC-BY-NC 4.0 license, unless otherwise stated. | |
Record name | Di-tert-butyl oxalate | |
Source | ChemIDplus | |
URL | https://pubchem.ncbi.nlm.nih.gov/substance/?source=chemidplus&sourceid=0000691645 | |
Description | ChemIDplus is a free, web search system that provides access to the structure and nomenclature authority files used for the identification of chemical substances cited in National Library of Medicine (NLM) databases, including the TOXNET system. | |
Record name | Di-tert-butyl oxalate | |
Source | EPA DSSTox | |
URL | https://comptox.epa.gov/dashboard/DTXSID20219157 | |
Description | DSSTox provides a high quality public chemistry resource for supporting improved predictive toxicology. | |
Record name | Di-tert-butyl oxalate | |
Source | European Chemicals Agency (ECHA) | |
URL | https://echa.europa.eu/substance-information/-/substanceinfo/100.010.659 | |
Description | The European Chemicals Agency (ECHA) is an agency of the European Union which is the driving force among regulatory authorities in implementing the EU's groundbreaking chemicals legislation for the benefit of human health and the environment as well as for innovation and competitiveness. | |
Explanation | Use of the information, documents and data from the ECHA website is subject to the terms and conditions of this Legal Notice, and subject to other binding limitations provided for under applicable law, the information, documents and data made available on the ECHA website may be reproduced, distributed and/or used, totally or in part, for non-commercial purposes provided that ECHA is acknowledged as the source: "Source: European Chemicals Agency, http://echa.europa.eu/". Such acknowledgement must be included in each copy of the material. ECHA permits and encourages organisations and individuals to create links to the ECHA website under the following cumulative conditions: Links can only be made to webpages that provide a link to the Legal Notice page. | |
Record name | Di-tert-butyl oxalate | |
Source | FDA Global Substance Registration System (GSRS) | |
URL | https://gsrs.ncats.nih.gov/ginas/app/beta/substances/GDT3TXT9FW | |
Description | The FDA Global Substance Registration System (GSRS) enables the efficient and accurate exchange of information on what substances are in regulated products. Instead of relying on names, which vary across regulatory domains, countries, and regions, the GSRS knowledge base makes it possible for substances to be defined by standardized, scientific descriptions. | |
Explanation | Unless otherwise noted, the contents of the FDA website (www.fda.gov), both text and graphics, are not copyrighted. They are in the public domain and may be republished, reprinted and otherwise used freely by anyone without the need to obtain permission from FDA. Credit to the U.S. Food and Drug Administration as the source is appreciated but not required. | |
Retrosynthesis Analysis
AI-Powered Synthesis Planning: Our tool employs the Template_relevance Pistachio, Template_relevance Bkms_metabolic, Template_relevance Pistachio_ringbreaker, Template_relevance Reaxys, Template_relevance Reaxys_biocatalysis model, leveraging a vast database of chemical reactions to predict feasible synthetic routes.
One-Step Synthesis Focus: Specifically designed for one-step synthesis, it provides concise and direct routes for your target compounds, streamlining the synthesis process.
Accurate Predictions: Utilizing the extensive PISTACHIO, BKMS_METABOLIC, PISTACHIO_RINGBREAKER, REAXYS, REAXYS_BIOCATALYSIS database, our tool offers high-accuracy predictions, reflecting the latest in chemical research and data.
Strategy Settings
Precursor scoring | Relevance Heuristic |
---|---|
Min. plausibility | 0.01 |
Model | Template_relevance |
Template Set | Pistachio/Bkms_metabolic/Pistachio_ringbreaker/Reaxys/Reaxys_biocatalysis |
Top-N result to add to graph | 6 |
Feasible Synthetic Routes
Q1: Why is Di-tert-butyl oxalate solid at room temperature, unlike other simple dialkyl oxalates?
A1: While most simple dialkyl oxalates are liquids at room temperature, this compound has a melting point of 343 K (70 °C). Research indicates that the presence of multiple C-H···O hydrogen bonds in its crystal structure contributes to this higher melting point. [, ] These interactions create stronger intermolecular forces, requiring more energy to break and transition from solid to liquid. Additionally, this compound exhibits a diminished entropic contribution (ΔSm) compared to other dialkyl oxalates, further increasing its melting point. []
Q2: Has the crystal structure of this compound been determined, and how does it compare to other dialkyl oxalates?
A2: Yes, the crystal structure of this compound has been determined using cryocrystallization techniques. [] This method allows for the crystallization of liquid compounds at low temperatures, enabling structural analysis. Comparing its structure to other dialkyl oxalates, such as diethyl, di-iso-propyl, and di-n-butyl oxalates, reveals a higher number of defined C-H···O interactions in this compound. [] These interactions are believed to play a key role in its higher melting point compared to its liquid counterparts.
Q3: Can this compound be used in synthetic reactions?
A3: Yes, this compound can act as a reagent in organic synthesis. For example, it reacts with hydrazine hydrate and 2-[(methylsulfanyl)methyl]oxirane to produce Di-tert-butyl 1-[2-hydroxy-3-(methylsulfanyl)propyl]hydrazine-1,2-dicarboxylate. [] This demonstrates its utility in forming complex molecules with potential applications in various fields.
Q4: What happens when Di-tert-butyl dicarbonate undergoes oxidative decarboxylation with thianthrene cation radical perchlorate?
A4: Studies have shown that Di-tert-butyl dicarbonate reacts with thianthrene cation radical perchlorate (Th+•ClO4−) in acetonitrile. [] This reaction leads to oxidative decarboxylation, breaking the C-O bond and yielding N-tert-butylacetamide, carbon dioxide, thianthrene-5-oxide, and thianthrene. The oxygen atom in thianthrene-5-oxide originates from Di-tert-butyl dicarbonate, indicating a unique pathway for this product's formation. []
Q5: Are there alternative synthetic routes to obtain erythro-β-fluoroaspartic acid and erythro-β-fluoroasparagine?
A5: Yes, research describes a method to synthesize erythro-β-fluoroaspartic acid and erythro-β-fluoroasparagine using this compound as a starting material. [] The synthesis involves reacting this compound with tert-butyl monofluoroacetate, followed by several steps including amination and reduction. This approach provides an alternative to conventional methods and highlights the versatility of this compound in synthesizing biologically relevant compounds. []
Disclaimer and Information on In-Vitro Research Products
Please be aware that all articles and product information presented on BenchChem are intended solely for informational purposes. The products available for purchase on BenchChem are specifically designed for in-vitro studies, which are conducted outside of living organisms. In-vitro studies, derived from the Latin term "in glass," involve experiments performed in controlled laboratory settings using cells or tissues. It is important to note that these products are not categorized as medicines or drugs, and they have not received approval from the FDA for the prevention, treatment, or cure of any medical condition, ailment, or disease. We must emphasize that any form of bodily introduction of these products into humans or animals is strictly prohibited by law. It is essential to adhere to these guidelines to ensure compliance with legal and ethical standards in research and experimentation.