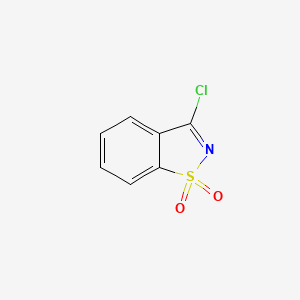
Pseudosaccharin chloride
Overview
Description
Pseudosaccharin chloride is a small molecule that belongs to the class of organic compounds known as benzothiazoles . These are organic compounds containing a benzene fused to a thiazole ring, which is a five-membered ring with four carbon atoms, one nitrogen atom, and one sulfur atom .
Synthesis Analysis
Pseudosaccharin amines were synthesized from saccharin either by the reaction of pseudosaccharin chloride with amines, or via thiosaccharin which was treated with amines yielding thiosaccharinates, and their reaction with glacial acetic acid . This route gave lower yields than the first way .
Molecular Structure Analysis
The molecular formula of Pseudosaccharin chloride is C7H4ClNO2S . It has an average mass of 201.62 Da and a mono-isotopic mass of 200.9651272 Da .
Scientific Research Applications
Environmental Science: Treatment of Oilfield Contaminants
Pseudosaccharin chloride has been studied for its potential in treating oilfield contaminants. Research indicates that compounds like Pseudosaccharin chloride can be used to induce the self-assembly of surfactants into micelle aggregates, which act as templates for creating adsorption materials with multi-level pore structures . These materials show promise in efficiently treating macromolecular contaminants such as sulfonated lignite, commonly found in oilfield wastewater.
Pharmacology: Drug Development
Although detailed pharmacological data for Pseudosaccharin chloride is not readily available, its structural class, benzothiazoles, are known for various therapeutic activities. Benzothiadiazine dioxides, a related scaffold, have applications ranging from antimicrobial to antihypertensive treatments . Pseudosaccharin chloride’s potential in drug development could be significant, given the wide range of activities exhibited by its chemical relatives.
Material Science: Adsorbent Development
The unique structural properties of Pseudosaccharin chloride make it a candidate for developing new adsorbent materials. Its ability to form hierarchical structures with a large specific surface area could be utilized in creating adsorbents for environmental applications, such as water purification and pollutant removal .
Analytical Chemistry: Ion-Exchange Chromatography
Pseudosaccharin chloride could be explored as a stationary phase in ion-exchange chromatography. Its chemical structure suggests potential for separating metal ions based on their chloride, fluoride, nitrate, or sulfate complexes . This application could be particularly useful in analytical methods for environmental and pharmaceutical analyses.
Medicinal Chemistry: Scaffold for Drug Synthesis
The benzothiadiazine dioxide ring system, to which Pseudosaccharin chloride belongs, has been utilized in synthesizing various pharmacologically active compounds. This includes antihypertensive, diuretic, and anticancer agents . Pseudosaccharin chloride could serve as a novel scaffold for developing new therapeutic agents.
Antimicrobial Research: Synthesis of Derivatives
Research into the antimicrobial properties of benzothiadiazine derivatives suggests that Pseudosaccharin chloride could be modified to enhance its antimicrobial activity. The synthesis of new derivatives could lead to the discovery of potent antimicrobial agents .
Mechanism of Action
properties
IUPAC Name |
3-chloro-1,2-benzothiazole 1,1-dioxide | |
---|---|---|
Source | PubChem | |
URL | https://pubchem.ncbi.nlm.nih.gov | |
Description | Data deposited in or computed by PubChem | |
InChI |
InChI=1S/C7H4ClNO2S/c8-7-5-3-1-2-4-6(5)12(10,11)9-7/h1-4H | |
Source | PubChem | |
URL | https://pubchem.ncbi.nlm.nih.gov | |
Description | Data deposited in or computed by PubChem | |
InChI Key |
VBEJRJPHNPIURV-UHFFFAOYSA-N | |
Source | PubChem | |
URL | https://pubchem.ncbi.nlm.nih.gov | |
Description | Data deposited in or computed by PubChem | |
Canonical SMILES |
C1=CC=C2C(=C1)C(=NS2(=O)=O)Cl | |
Source | PubChem | |
URL | https://pubchem.ncbi.nlm.nih.gov | |
Description | Data deposited in or computed by PubChem | |
Molecular Formula |
C7H4ClNO2S | |
Source | PubChem | |
URL | https://pubchem.ncbi.nlm.nih.gov | |
Description | Data deposited in or computed by PubChem | |
DSSTOX Substance ID |
DTXSID00205255 | |
Record name | Pseudosaccharin chloride | |
Source | EPA DSSTox | |
URL | https://comptox.epa.gov/dashboard/DTXSID00205255 | |
Description | DSSTox provides a high quality public chemistry resource for supporting improved predictive toxicology. | |
Molecular Weight |
201.63 g/mol | |
Source | PubChem | |
URL | https://pubchem.ncbi.nlm.nih.gov | |
Description | Data deposited in or computed by PubChem | |
Product Name |
Pseudosaccharin chloride | |
CAS RN |
567-19-1 | |
Record name | Pseudosaccharin chloride | |
Source | ChemIDplus | |
URL | https://pubchem.ncbi.nlm.nih.gov/substance/?source=chemidplus&sourceid=0000567191 | |
Description | ChemIDplus is a free, web search system that provides access to the structure and nomenclature authority files used for the identification of chemical substances cited in National Library of Medicine (NLM) databases, including the TOXNET system. | |
Record name | Pseudosaccharin chloride | |
Source | DrugBank | |
URL | https://www.drugbank.ca/drugs/DB14628 | |
Description | The DrugBank database is a unique bioinformatics and cheminformatics resource that combines detailed drug (i.e. chemical, pharmacological and pharmaceutical) data with comprehensive drug target (i.e. sequence, structure, and pathway) information. | |
Explanation | Creative Common's Attribution-NonCommercial 4.0 International License (http://creativecommons.org/licenses/by-nc/4.0/legalcode) | |
Record name | Pseudosaccharin chloride | |
Source | DTP/NCI | |
URL | https://dtp.cancer.gov/dtpstandard/servlet/dwindex?searchtype=NSC&outputformat=html&searchlist=49757 | |
Description | The NCI Development Therapeutics Program (DTP) provides services and resources to the academic and private-sector research communities worldwide to facilitate the discovery and development of new cancer therapeutic agents. | |
Explanation | Unless otherwise indicated, all text within NCI products is free of copyright and may be reused without our permission. Credit the National Cancer Institute as the source. | |
Record name | Pseudosaccharin chloride | |
Source | EPA DSSTox | |
URL | https://comptox.epa.gov/dashboard/DTXSID00205255 | |
Description | DSSTox provides a high quality public chemistry resource for supporting improved predictive toxicology. | |
Record name | 3-chloro-1,2-benzothiazole 1,1-dioxide | |
Source | European Chemicals Agency (ECHA) | |
URL | https://echa.europa.eu/information-on-chemicals | |
Description | The European Chemicals Agency (ECHA) is an agency of the European Union which is the driving force among regulatory authorities in implementing the EU's groundbreaking chemicals legislation for the benefit of human health and the environment as well as for innovation and competitiveness. | |
Explanation | Use of the information, documents and data from the ECHA website is subject to the terms and conditions of this Legal Notice, and subject to other binding limitations provided for under applicable law, the information, documents and data made available on the ECHA website may be reproduced, distributed and/or used, totally or in part, for non-commercial purposes provided that ECHA is acknowledged as the source: "Source: European Chemicals Agency, http://echa.europa.eu/". Such acknowledgement must be included in each copy of the material. ECHA permits and encourages organisations and individuals to create links to the ECHA website under the following cumulative conditions: Links can only be made to webpages that provide a link to the Legal Notice page. | |
Record name | PSEUDOSACCHARIN CHLORIDE | |
Source | FDA Global Substance Registration System (GSRS) | |
URL | https://gsrs.ncats.nih.gov/ginas/app/beta/substances/1AYD6UVV3F | |
Description | The FDA Global Substance Registration System (GSRS) enables the efficient and accurate exchange of information on what substances are in regulated products. Instead of relying on names, which vary across regulatory domains, countries, and regions, the GSRS knowledge base makes it possible for substances to be defined by standardized, scientific descriptions. | |
Explanation | Unless otherwise noted, the contents of the FDA website (www.fda.gov), both text and graphics, are not copyrighted. They are in the public domain and may be republished, reprinted and otherwise used freely by anyone without the need to obtain permission from FDA. Credit to the U.S. Food and Drug Administration as the source is appreciated but not required. | |
Synthesis routes and methods I
Procedure details
Synthesis routes and methods II
Procedure details
Synthesis routes and methods III
Procedure details
Synthesis routes and methods IV
Procedure details
Retrosynthesis Analysis
AI-Powered Synthesis Planning: Our tool employs the Template_relevance Pistachio, Template_relevance Bkms_metabolic, Template_relevance Pistachio_ringbreaker, Template_relevance Reaxys, Template_relevance Reaxys_biocatalysis model, leveraging a vast database of chemical reactions to predict feasible synthetic routes.
One-Step Synthesis Focus: Specifically designed for one-step synthesis, it provides concise and direct routes for your target compounds, streamlining the synthesis process.
Accurate Predictions: Utilizing the extensive PISTACHIO, BKMS_METABOLIC, PISTACHIO_RINGBREAKER, REAXYS, REAXYS_BIOCATALYSIS database, our tool offers high-accuracy predictions, reflecting the latest in chemical research and data.
Strategy Settings
Precursor scoring | Relevance Heuristic |
---|---|
Min. plausibility | 0.01 |
Model | Template_relevance |
Template Set | Pistachio/Bkms_metabolic/Pistachio_ringbreaker/Reaxys/Reaxys_biocatalysis |
Top-N result to add to graph | 6 |
Feasible Synthetic Routes
Disclaimer and Information on In-Vitro Research Products
Please be aware that all articles and product information presented on BenchChem are intended solely for informational purposes. The products available for purchase on BenchChem are specifically designed for in-vitro studies, which are conducted outside of living organisms. In-vitro studies, derived from the Latin term "in glass," involve experiments performed in controlled laboratory settings using cells or tissues. It is important to note that these products are not categorized as medicines or drugs, and they have not received approval from the FDA for the prevention, treatment, or cure of any medical condition, ailment, or disease. We must emphasize that any form of bodily introduction of these products into humans or animals is strictly prohibited by law. It is essential to adhere to these guidelines to ensure compliance with legal and ethical standards in research and experimentation.