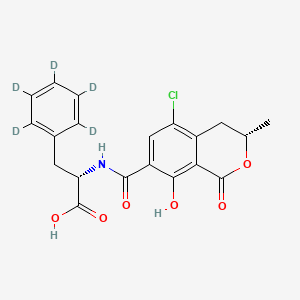
3-epi-Ochratoxin A-d5
- Click on QUICK INQUIRY to receive a quote from our team of experts.
- With the quality product at a COMPETITIVE price, you can focus more on your research.
Overview
Description
3-epi-Ochratoxin A-d5: is a deuterium-labeled analog of 3-epi-Ochratoxin A, a mycotoxin produced by certain species of Aspergillus and Penicillium fungi. This compound is primarily used in scientific research to study the toxicological effects and metabolic pathways of ochratoxins. The deuterium labeling allows for precise tracking and quantification in various analytical methods.
Preparation Methods
Synthetic Routes and Reaction Conditions: The synthesis of 3-epi-Ochratoxin A-d5 involves the incorporation of deuterium atoms into the molecular structure of 3-epi-Ochratoxin A. This is typically achieved through a series of chemical reactions that replace hydrogen atoms with deuterium. The process often involves the use of deuterated reagents and solvents under controlled conditions to ensure the selective incorporation of deuterium.
Industrial Production Methods: Industrial production of this compound is generally carried out in specialized laboratories equipped with advanced chemical synthesis and purification technologies. The process includes:
Fermentation: Culturing Aspergillus or Penicillium species to produce ochratoxins.
Extraction: Isolating ochratoxins from the culture medium using solvents like methanol and water.
Deuteration: Introducing deuterium atoms through chemical reactions.
Purification: Using techniques such as liquid-liquid extraction and chromatography to obtain high-purity this compound
Chemical Reactions Analysis
Types of Reactions: 3-epi-Ochratoxin A-d5 undergoes various chemical reactions, including:
Oxidation: Conversion to more oxidized forms.
Reduction: Reduction of functional groups.
Substitution: Replacement of functional groups with other atoms or groups.
Common Reagents and Conditions:
Oxidation: Reagents like hydrogen peroxide or potassium permanganate under acidic or basic conditions.
Reduction: Reagents such as sodium borohydride or lithium aluminum hydride.
Substitution: Halogenating agents or nucleophiles under specific conditions.
Major Products: The major products formed from these reactions depend on the specific reagents and conditions used. For example, oxidation may yield hydroxylated derivatives, while reduction could produce dechlorinated forms .
Scientific Research Applications
3-epi-Ochratoxin A-d5 is extensively used in scientific research due to its labeled nature, which facilitates detailed studies on:
Chemistry: Understanding the chemical properties and reactivity of ochratoxins.
Biology: Investigating the biological effects and metabolic pathways in living organisms.
Medicine: Studying the toxicological impacts and potential therapeutic interventions.
Industry: Monitoring and controlling ochratoxin contamination in food and agricultural products
Mechanism of Action
The mechanism of action of 3-epi-Ochratoxin A-d5 involves its interaction with various molecular targets and pathways:
Protein Synthesis Inhibition: It inhibits protein synthesis by interfering with ribosomal function.
Oxidative Stress Induction: It induces oxidative stress by generating reactive oxygen species.
DNA Adduct Formation: It forms adducts with DNA, leading to genotoxic effects.
Apoptosis and Necrosis: It triggers cell death through apoptosis and necrosis pathways
Comparison with Similar Compounds
Ochratoxin A-d5: Another deuterium-labeled ochratoxin used for similar research purposes.
3-epi-Ochratoxin C-d5: A related compound with a different functional group.
Ochratoxin B: A non-chlorinated analog of ochratoxin A.
Uniqueness: 3-epi-Ochratoxin A-d5 is unique due to its specific deuterium labeling, which allows for precise tracking in metabolic studies. Its structural similarity to 3-epi-Ochratoxin A makes it an ideal analog for studying the toxicological effects and metabolic pathways of ochratoxins .
Properties
Molecular Formula |
C20H18ClNO6 |
---|---|
Molecular Weight |
408.8 g/mol |
IUPAC Name |
(2S)-2-[[(3S)-5-chloro-8-hydroxy-3-methyl-1-oxo-3,4-dihydroisochromene-7-carbonyl]amino]-3-(2,3,4,5,6-pentadeuteriophenyl)propanoic acid |
InChI |
InChI=1S/C20H18ClNO6/c1-10-7-12-14(21)9-13(17(23)16(12)20(27)28-10)18(24)22-15(19(25)26)8-11-5-3-2-4-6-11/h2-6,9-10,15,23H,7-8H2,1H3,(H,22,24)(H,25,26)/t10-,15-/m0/s1/i2D,3D,4D,5D,6D |
InChI Key |
RWQKHEORZBHNRI-JYRICJBVSA-N |
Isomeric SMILES |
[2H]C1=C(C(=C(C(=C1[2H])[2H])C[C@@H](C(=O)O)NC(=O)C2=CC(=C3C[C@@H](OC(=O)C3=C2O)C)Cl)[2H])[2H] |
Canonical SMILES |
CC1CC2=C(C=C(C(=C2C(=O)O1)O)C(=O)NC(CC3=CC=CC=C3)C(=O)O)Cl |
Origin of Product |
United States |
Disclaimer and Information on In-Vitro Research Products
Please be aware that all articles and product information presented on BenchChem are intended solely for informational purposes. The products available for purchase on BenchChem are specifically designed for in-vitro studies, which are conducted outside of living organisms. In-vitro studies, derived from the Latin term "in glass," involve experiments performed in controlled laboratory settings using cells or tissues. It is important to note that these products are not categorized as medicines or drugs, and they have not received approval from the FDA for the prevention, treatment, or cure of any medical condition, ailment, or disease. We must emphasize that any form of bodily introduction of these products into humans or animals is strictly prohibited by law. It is essential to adhere to these guidelines to ensure compliance with legal and ethical standards in research and experimentation.