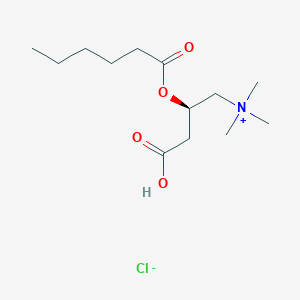
Hexanoyl-L-carnitine chloride
Overview
Description
Hexanoyl-L-carnitine chloride (CAS 162067-53-0) is a medium-chain acylcarnitine derivative composed of L-carnitine esterified with a hexanoyl (C6:0) fatty acid group. Its molecular formula is C₁₃H₂₆ClNO₄, with a molecular weight of 295.8 g/mol . Structurally, it features a quaternary ammonium group, a carboxylate moiety, and a hexanoyl-esterified hydroxyl group, enabling its role in mitochondrial fatty acid transport .
Preparation Methods
Synthetic Routes and Reaction Conditions: Hexanoyl-L-carnitine (chloride) can be synthesized through the esterification of L-carnitine with hexanoic acid. The reaction typically involves the use of a coupling agent such as dicyclohexylcarbodiimide (DCC) and a catalyst like 4-dimethylaminopyridine (DMAP) in an organic solvent such as dichloromethane . The reaction is carried out under anhydrous conditions to prevent hydrolysis of the ester bond.
Industrial Production Methods: In an industrial setting, the production of Hexanoyl-L-carnitine (chloride) may involve the use of continuous flow reactors to optimize reaction conditions and improve yield. The process includes the purification of the product through crystallization or chromatography to achieve high purity levels .
Chemical Reactions Analysis
Types of Reactions: Hexanoyl-L-carnitine (chloride) undergoes various chemical reactions, including:
Oxidation: It can be oxidized to form carboxylic acids.
Reduction: Reduction reactions can convert it into alcohols.
Substitution: Nucleophilic substitution reactions can replace the chloride ion with other nucleophiles.
Common Reagents and Conditions:
Oxidation: Common oxidizing agents include potassium permanganate (KMnO₄) and chromium trioxide (CrO₃).
Reduction: Reducing agents such as lithium aluminum hydride (LiAlH₄) and sodium borohydride (NaBH₄) are used.
Substitution: Nucleophiles like hydroxide ions (OH⁻) and amines are commonly used.
Major Products:
Oxidation: Carboxylic acids.
Reduction: Alcohols.
Substitution: Various substituted carnitine derivatives.
Scientific Research Applications
Hexanoyl-L-carnitine chloride is a medium-chain acylcarnitine derivative that plays a vital role in energy metabolism and has diverse applications in scientific research. Its unique properties, stemming from its hexanoyl group, make it particularly effective in specific metabolic contexts.
Scientific Research Applications
Biochemical Research this compound is used as a substrate in studies involving fatty acid metabolism. It plays a crucial role in transporting long-chain fatty acids into the mitochondria for beta-oxidation, enhancing mitochondrial function, and potentially treating metabolic disorders related to fatty acid utilization .
Clinical Research This compound has been investigated for potential therapeutic applications. Studies suggest it has antioxidant properties and can modulate cellular signaling pathways.
Metabolic Studies this compound is used to study fatty acid metabolism, helping researchers understand energy production and storage in cells . It influences the activity of enzymes involved in lipid metabolism and positively affects mitochondrial function.
Cardiovascular Health It is used in investigations related to heart function and health, particularly how fatty acids influence cardiac metabolism and performance . Carnitine, in general, plays a role in transporting long-chain fatty acids in myocardial mitochondria, where they are metabolized for energy . It also moderates oxidative stress and might decrease markers of inflammation .
Weight Management Researchers are exploring its potential in weight loss and obesity studies, as it may aid in the transportation of fatty acids into mitochondria for energy use .
Neuroprotective Research The compound is studied for its effects on brain health, particularly in neurodegenerative diseases, due to its role in cellular energy metabolism . Carnitine might be conditionally essential in individuals with Alzheimer’s disease because it may support acetylcholine synthesis and help remove toxic compounds to alleviate mitochondrial dysfunction .
Pharmaceutical Development It is a key ingredient in formulations aimed at enhancing drug delivery systems, particularly for lipid-based therapies, improving bioavailability and efficacy .
Comparative Analysis of Acylcarnitines
This compound shares structural similarities with other acylcarnitines but has unique properties because of its specific hexanoyl group.
Compound Name | Chemical Formula | Unique Features |
---|---|---|
Acetyl-L-carnitine | C₈H₁₅NO₄ | Shorter acyl chain; used for cognitive enhancement |
Octanoyl-L-carnitine | C₉H₁₉NO₄ | Longer acyl chain; impacts energy metabolism differently |
Palmitoyl-L-carnitine | C₁₃H₂₅NO₄ | Even longer acyl chain; associated with different metabolic profiles |
Case Studies and Research Findings
- MCAD Deficiency Hexanoylglycine and suberylglycine were synthesized to study medium-chain acyl-coA dehydrogenase (MCAD) deficiency . A combination therapy of reduced dietary fat and L-carnitine supplementation has shown positive outcomes in new cases .
- Taxane-Induced Neuropathy A randomized, double-blind, placebo-controlled trial of acetyl-L-carnitine for the prevention of taxane-induced neuropathy in women undergoing adjuvant breast cancer treatment showed that patients receiving acetyl-L-carnitine (ALC) were more likely to have a > 5-point decrease in FACT-NTX scores . However, grade 3 to 4 neurotoxicity was more frequent in the ALC arm .
- Metabolic Syndrome Biomarkers An LC-MS/MS method was used to quantify acylcarnitine species in streptozotocin-treated diabetic mice, identifying significantly increased concentrations of acylcarnitines derived from branched-chain amino acid degradation and of odd-numbered straight-chain species, proposed as potential biomarkers for metabolic syndrome .
- Cardiovascular Disease A meta-analysis of clinical trials found that L-carnitine significantly reduced rates of all-cause mortality, ventricular arrhythmias, and new-onset angina in adults with acute myocardial infarction but did not affect the risk of heart failure or myocardial reinfarction . Another meta-analysis found that L-carnitine supplements improved left ventricular ejection fraction, stroke volume, and cardiac output in adults with chronic heart failure .
Mechanism of Action
Hexanoyl-L-carnitine (chloride) facilitates the transport of fatty acids into the mitochondria, where they undergo β-oxidation to produce energy. This process involves the conversion of fatty acids into acyl-CoA derivatives, which are then transported across the mitochondrial membrane by carnitine acyltransferase enzymes . The compound’s molecular targets include carnitine palmitoyltransferase I (CPT1) and carnitine palmitoyltransferase II (CPT2), which are key enzymes in the carnitine shuttle pathway .
Comparison with Similar Compounds
Physicochemical Properties :
- Melting Point : >166°C (decomposition)
- Solubility: Slightly soluble in water, DMSO, and methanol .
- Storage : Stable at 0°C in dry, dark conditions .
Acylcarnitines are classified by fatty acid chain length (short: C2–C5; medium: C6–C12; long: >C12). Below is a systematic comparison:
Short-Chain Acylcarnitines
Valeryl-L-carnitine chloride (C5:0)
- Molecular Weight : ~240.7 g/mol .
- Function : Inhibits intestinal carnitine/glycine transport at high concentrations .
- Applications : Studied in metabolic disease models .
Acetyl-L-carnitine chloride (C2:0)
- Molecular Weight : 239.7 g/mol .
- Function : Transports acetyl groups into mitochondria; neuroprotective and cognitive-enhancing effects .
- Key Difference: Unlike hexanoyl, acetyl derivatives are critical in acetylcholine synthesis and neurodegenerative disease research .
Medium-Chain Acylcarnitines
Hexanoyl-L-carnitine chloride (C6:0)
- Solubility : Moderate in polar solvents due to balanced hydrophobicity .
- Metabolic Role : Optimized for medium-chain fatty acid oxidation .
Heptanoyl-L-carnitine chloride (C7:0)
- Molecular Weight : ~309.8 g/mol .
- Function : Shares β-oxidation roles but with longer retention in lipid membranes due to increased hydrophobicity .
Octanoyl-L-carnitine chloride (C8:0)
- Molecular Weight : ~323.9 g/mol .
- Applications : Used in studies of lipid metabolism and insulin resistance .
Long-Chain Acylcarnitines
Lauroyl-L-carnitine chloride (C12:0)
- Molecular Weight : 379.96 g/mol .
- Function : Surfactant properties enable membrane permeabilization; linked to insulin signaling and glycine transport inhibition .
- Solubility : Poor water solubility due to long hydrophobic tail .
Palmitoyl-L-carnitine chloride (C16:0)
Comparative Data Table
Key Research Findings
Hexanoyl vs. Acetyl Derivatives: Hexanoyl-L-carnitine is more efficient in transporting medium-chain fatty acids, while acetyl-L-carnitine is pivotal in acetyl group metabolism and neurological health .
Chain Length and Solubility: Water solubility decreases with increasing acyl chain length. Hexanoyl (C6) strikes a balance between mitochondrial uptake and solubility .
Therapeutic Potential: Long-chain acylcarnitines (e.g., palmitoyl) show dual roles in cytoprotection and cytotoxicity, whereas hexanoyl’s medium chain minimizes cellular toxicity .
Biological Activity
Hexanoyl-L-carnitine chloride is a medium-chain acylcarnitine derivative that has garnered attention for its significant biological activity, particularly in the context of energy metabolism. This compound is characterized by a hexanoyl group attached to the carnitine molecule, with a chemical formula of C₁₃H₂₆ClNO₄ and a molecular weight of 295.8 g/mol. Its role as an intermediate in lipid metabolism makes it a valuable subject of study in metabolic research.
This compound plays a crucial role in the transport of long-chain fatty acids into mitochondria for beta-oxidation, which is essential for energy production in cells. This compound enhances mitochondrial function and has been implicated in various metabolic disorders associated with fatty acid utilization. Notably, it may also exhibit antioxidant properties and influence cellular signaling pathways related to energy expenditure and oxidative stress responses .
Research Findings
Recent studies have explored the biological activity of this compound through various methodologies, including mass spectrometry and metabolic profiling. For instance, a study demonstrated its impact on lipid metabolism by analyzing acylcarnitines in patients with metabolic disorders, revealing alterations in their concentrations that correlated with disease states .
Case Studies and Clinical Applications
- Metabolic Disorders : Research indicates that supplementation with this compound can aid in the management of conditions like medium-chain acyl-CoA dehydrogenase deficiency. In clinical settings, patients receiving this compound showed improved metabolic profiles, including normalized levels of specific acylcarnitines over time .
- Energy Metabolism : In animal models, particularly PPARalpha(-/-) mice, oral supplementation with L-carnitine (from which Hexanoyl-L-carnitine is derived) resulted in partial metabolic recovery and improved fatty acid oxidation profiles. This highlights the potential therapeutic applications of this compound in enhancing energy metabolism .
Comparative Analysis
The following table summarizes the key characteristics and comparative biological activities of various acylcarnitines, including this compound:
Compound Name | Chemical Formula | Unique Features | Biological Activity |
---|---|---|---|
Hexanoyl-L-carnitine | C₁₃H₂₆ClNO₄ | Medium-chain length; rapid metabolism | Enhances mitochondrial function |
Acetyl-L-carnitine | C₈H₁₅NO₄ | Shorter acyl chain; cognitive enhancement applications | Neuroprotective effects |
Octanoyl-L-carnitine | C₉H₁₉NO₄ | Longer acyl chain; different energy metabolism impact | Involved in lipid metabolism |
Palmitoyl-L-carnitine | C₁₃H₂₅NO₄ | Even longer acyl chain; distinct metabolic profiles | Associated with different metabolic pathways |
Q & A
Basic Research Questions
Q. What validated analytical methods are recommended for quantifying Hexanoyl-L-carnitine chloride in biological samples?
- Methodological Answer : High-performance liquid chromatography coupled with electrospray ionization tandem mass spectrometry (HPLC-ESI-MS/MS) is the gold standard for quantifying this compound in dried blood spots and human plasma. This method ensures high sensitivity (detection limits < 0.1 µM) and specificity, avoiding derivatization steps that may alter analyte integrity . Calibration curves should be validated using isotopically labeled internal standards (e.g., Hexanoyl-L-carnitine-d3 hydrochloride) to correct for matrix effects .
Q. How does this compound differ functionally from other medium-chain acyl carnitines (e.g., Decanoyl-L-carnitine)?
- Methodological Answer : Comparative studies using in vitro β-oxidation assays (e.g., isolated liver mitochondria) reveal that chain length impacts metabolic flux. Hexanoyl-L-carnitine (C6) exhibits faster transport into mitochondria compared to Decanoyl-L-carnitine (C10) due to reduced steric hindrance, as shown by kinetic parameters (Km = 12 µM vs. 25 µM). However, C10 derivatives generate higher ATP yields in prolonged assays .
Q. What experimental models are suitable for studying this compound’s role in lipid metabolism?
- Methodological Answer : Primary hepatocyte cultures and genetically modified rodent models (e.g., CPT1A-deficient mice) are optimal. Hepatocytes treated with 100 µM this compound show a 40% increase in fatty acid oxidation rates, measured via radiolabeled [14C]-palmitate incorporation into CO₂. In vivo models require plasma concentration monitoring via LC-MS to avoid supraphysiological dosing artifacts .
Advanced Research Questions
Q. How can researchers resolve contradictory data on this compound’s antioxidant effects in metabolic studies?
- Methodological Answer : Discrepancies arise from assay choice. For example:
- Lipid peroxidation assays (TBARS method) show 30% reduction in oxidative markers at 50 µM .
- ROS-sensitive fluorescent probes (e.g., DCFH-DA) report no significant effect in myotubes.
- Resolution : Use orthogonal methods (e.g., electron paramagnetic resonance spectroscopy) to directly measure free radical scavenging. Context-dependent effects (cell type, baseline oxidative stress) must be controlled .
Q. What synthesis strategies improve the purity of this compound for isotopic labeling studies?
- Methodological Answer : Acylation of L-carnitine with hexanoyl chloride under anhydrous conditions (pH 8.5–9.0, 4°C) minimizes hydrolysis. Purification via reversed-phase HPLC (C18 column, 0.1% TFA in acetonitrile/water gradient) achieves >98% purity. Deuterated analogs (e.g., Hexanoyl-L-carnitine-d3) require strict exclusion of protic solvents to prevent H/D exchange .
Q. How does this compound interact with mitochondrial membrane proteins during β-oxidation?
- Methodological Answer : Surface plasmon resonance (SPR) assays with immobilized carnitine palmitoyltransferase 1 (CPT1) reveal a binding affinity (Kd) of 8.2 ± 1.3 µM. Molecular dynamics simulations suggest the hexanoyl moiety stabilizes hydrophobic interactions with CPT1’s acyl-CoA binding pocket. Mutagenesis studies (e.g., Arg498Ala) disrupt binding, confirming electrostatic contributions .
Q. Data Analysis and Experimental Design
Q. What statistical approaches are recommended for analyzing dose-dependent effects of this compound in cell viability assays?
- Methodological Answer : Nonlinear regression (e.g., four-parameter logistic model) calculates EC₅₀ values. For high-throughput screens, normalized viability data should undergo robust Z-score transformation to mitigate plate-to-plate variability. Confounders (e.g., serum lipid content) require stratification .
Q. How can researchers validate this compound as a biomarker for metabolic disorders?
- Methodological Answer : Multi-cohort LC-MS/MS profiling (n > 500 samples) with ROC curve analysis identifies optimal cutoff values (e.g., 0.8 µM in plasma for medium-chain acyl-CoA dehydrogenase deficiency). Cross-validation against genetic testing (ACADM mutations) ensures specificity. Pre-analytical variables (e.g., fasting status) must be standardized .
Q. Tables
Table 1. Key Physicochemical Properties of this compound
Property | Value | Reference |
---|---|---|
Molecular Weight | 295.80 g/mol | |
Solubility (25°C) | 50 mg/mL in water | |
Stability | Stable at 2–8°C for 24 months | |
HPLC Retention Time (C18) | 6.2 ± 0.3 min |
Table 2. Comparison of Acyl Carnitines in Metabolic Pathways
Properties
IUPAC Name |
[(2R)-3-carboxy-2-hexanoyloxypropyl]-trimethylazanium;chloride | |
---|---|---|
Source | PubChem | |
URL | https://pubchem.ncbi.nlm.nih.gov | |
Description | Data deposited in or computed by PubChem | |
InChI |
InChI=1S/C13H25NO4.ClH/c1-5-6-7-8-13(17)18-11(9-12(15)16)10-14(2,3)4;/h11H,5-10H2,1-4H3;1H/t11-;/m1./s1 | |
Source | PubChem | |
URL | https://pubchem.ncbi.nlm.nih.gov | |
Description | Data deposited in or computed by PubChem | |
InChI Key |
DTHGTKVOSRYXOK-RFVHGSKJSA-N | |
Source | PubChem | |
URL | https://pubchem.ncbi.nlm.nih.gov | |
Description | Data deposited in or computed by PubChem | |
Canonical SMILES |
CCCCCC(=O)OC(CC(=O)O)C[N+](C)(C)C.[Cl-] | |
Source | PubChem | |
URL | https://pubchem.ncbi.nlm.nih.gov | |
Description | Data deposited in or computed by PubChem | |
Isomeric SMILES |
CCCCCC(=O)O[C@H](CC(=O)O)C[N+](C)(C)C.[Cl-] | |
Source | PubChem | |
URL | https://pubchem.ncbi.nlm.nih.gov | |
Description | Data deposited in or computed by PubChem | |
Molecular Formula |
C13H26ClNO4 | |
Source | PubChem | |
URL | https://pubchem.ncbi.nlm.nih.gov | |
Description | Data deposited in or computed by PubChem | |
Molecular Weight |
295.80 g/mol | |
Source | PubChem | |
URL | https://pubchem.ncbi.nlm.nih.gov | |
Description | Data deposited in or computed by PubChem | |
Retrosynthesis Analysis
AI-Powered Synthesis Planning: Our tool employs the Template_relevance Pistachio, Template_relevance Bkms_metabolic, Template_relevance Pistachio_ringbreaker, Template_relevance Reaxys, Template_relevance Reaxys_biocatalysis model, leveraging a vast database of chemical reactions to predict feasible synthetic routes.
One-Step Synthesis Focus: Specifically designed for one-step synthesis, it provides concise and direct routes for your target compounds, streamlining the synthesis process.
Accurate Predictions: Utilizing the extensive PISTACHIO, BKMS_METABOLIC, PISTACHIO_RINGBREAKER, REAXYS, REAXYS_BIOCATALYSIS database, our tool offers high-accuracy predictions, reflecting the latest in chemical research and data.
Strategy Settings
Precursor scoring | Relevance Heuristic |
---|---|
Min. plausibility | 0.01 |
Model | Template_relevance |
Template Set | Pistachio/Bkms_metabolic/Pistachio_ringbreaker/Reaxys/Reaxys_biocatalysis |
Top-N result to add to graph | 6 |
Feasible Synthetic Routes
Disclaimer and Information on In-Vitro Research Products
Please be aware that all articles and product information presented on BenchChem are intended solely for informational purposes. The products available for purchase on BenchChem are specifically designed for in-vitro studies, which are conducted outside of living organisms. In-vitro studies, derived from the Latin term "in glass," involve experiments performed in controlled laboratory settings using cells or tissues. It is important to note that these products are not categorized as medicines or drugs, and they have not received approval from the FDA for the prevention, treatment, or cure of any medical condition, ailment, or disease. We must emphasize that any form of bodily introduction of these products into humans or animals is strictly prohibited by law. It is essential to adhere to these guidelines to ensure compliance with legal and ethical standards in research and experimentation.