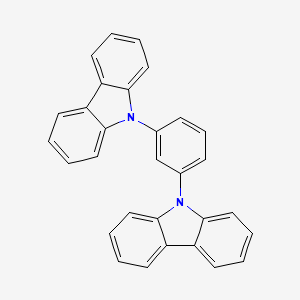
1,3-Bis(N-carbazolyl)benzene
Overview
Description
1,3-Bis(N-carbazolyl)benzene is an organic compound consisting of a central benzene ring connected to two carbazolyl groups. It is commonly used as a hole transporting material in organic light-emitting diodes (OLEDs) due to its high thermal stability and excellent charge transport properties . The carbazolyl groups in the molecule help to facilitate the movement of positive charge carriers (holes) within the device, leading to efficient light emission .
Biochemical Analysis
Biochemical Properties
1,3-Bis(N-carbazolyl)benzene plays a significant role in biochemical reactions, particularly in the context of organic electronics. It interacts with various enzymes, proteins, and other biomolecules. For instance, it has been observed to increase the photoluminescence internal quantum yield of blue emitters like FIrpic when doped into the wide energy gap host of this compound . The nature of these interactions involves the transfer of energy and electrons, which enhances the efficiency of the emitting materials.
Cellular Effects
The effects of this compound on various types of cells and cellular processes are profound. It influences cell function by affecting cell signaling pathways, gene expression, and cellular metabolism. For example, in OLED applications, this compound has been shown to facilitate efficient energy transfer, which can impact cellular processes related to energy metabolism and signaling . This compound’s ability to modulate these pathways makes it a valuable tool in studying cellular functions and developing new biochemical applications.
Molecular Mechanism
The molecular mechanism of this compound involves its interaction with biomolecules at the molecular level. It exerts its effects through binding interactions with enzymes and proteins, leading to enzyme inhibition or activation and changes in gene expression. The high triplet energy and deep HOMO levels of this compound enable it to act as an efficient host material, facilitating energy transfer and enhancing the photoluminescence of doped materials . These interactions are crucial for its role in biochemical reactions and organic electronics.
Temporal Effects in Laboratory Settings
In laboratory settings, the effects of this compound change over time. Studies have shown that this compound exhibits stability and minimal degradation over extended periods, making it suitable for long-term applications . The long-term effects on cellular function observed in in vitro and in vivo studies indicate that this compound maintains its efficacy and stability, ensuring consistent performance in biochemical applications.
Dosage Effects in Animal Models
The effects of this compound vary with different dosages in animal models. At lower dosages, it has been observed to enhance cellular functions without causing adverse effects. At higher dosages, there may be threshold effects, including potential toxicity or adverse reactions . These findings highlight the importance of optimizing dosage levels to achieve the desired biochemical effects while minimizing potential risks.
Metabolic Pathways
This compound is involved in various metabolic pathways, interacting with enzymes and cofactors. It affects metabolic flux and metabolite levels, contributing to its role in biochemical reactions. The compound’s interactions with metabolic enzymes facilitate its incorporation into metabolic pathways, enhancing its efficacy in biochemical applications .
Transport and Distribution
The transport and distribution of this compound within cells and tissues involve specific transporters and binding proteins. These interactions influence its localization and accumulation, ensuring that the compound reaches its target sites effectively . The efficient transport and distribution mechanisms are crucial for its role in biochemical reactions and organic electronics.
Subcellular Localization
This compound exhibits specific subcellular localization, which affects its activity and function. Targeting signals and post-translational modifications direct it to specific compartments or organelles, enhancing its efficacy in biochemical reactions . The precise subcellular localization ensures that this compound interacts with the appropriate biomolecules, facilitating its role in various biochemical processes.
Preparation Methods
Synthetic Routes and Reaction Conditions
1,3-Bis(N-carbazolyl)benzene can be synthesized through a palladium-catalyzed cross-coupling reaction between carbazole and 1,3-dibromobenzene . The reaction typically involves the use of a palladium catalyst, such as palladium acetate, and a base, such as potassium carbonate, in a solvent like toluene. The reaction is carried out under an inert atmosphere, such as nitrogen or argon, at elevated temperatures (around 100-120°C) for several hours .
Industrial Production Methods
In industrial settings, the production of this compound may involve similar synthetic routes but on a larger scale. The use of continuous flow reactors and optimized reaction conditions can enhance the efficiency and yield of the compound. Additionally, purification techniques such as recrystallization and sublimation are employed to obtain high-purity this compound .
Chemical Reactions Analysis
Types of Reactions
1,3-Bis(N-carbazolyl)benzene undergoes various chemical reactions, including:
Common Reagents and Conditions
Oxidation: Potassium permanganate in an acidic medium.
Reduction: Lithium aluminum hydride in anhydrous ether.
Substitution: Electrophilic reagents like halogens or nitro groups in the presence of a catalyst.
Major Products Formed
Oxidation: Formation of oxidized derivatives of this compound.
Reduction: Formation of reduced derivatives with altered electronic properties.
Scientific Research Applications
1,3-Bis(N-carbazolyl)benzene has a wide range of scientific research applications, including:
Organic Light-Emitting Diodes (OLEDs): Used as a host material to improve the efficiency and stability of OLEDs.
OLED Displays: Employed in the fabrication of high-performance OLED displays for TVs, smartphones, and wearable devices.
Hole-Transport Material: Functions as a hole-transport material in OLED devices, enabling efficient hole injection and transport.
Organic Photovoltaics: Enhances charge transport in organic photovoltaic devices, improving solar cell efficiency.
Organic Field-Effect Transistors (OFETs): Used as a semiconductor material in OFETs for flexible and lightweight electronic devices.
Organic Photoconductors: Essential component in photocopiers, laser printers, and other optical devices.
Sensors: Explored for use in chemical and biological sensors due to its high sensitivity and selectivity.
Mechanism of Action
The mechanism of action of 1,3-Bis(N-carbazolyl)benzene in OLEDs involves its role as a host material that facilitates the transport of positive charge carriers (holes) within the device . The carbazolyl groups in the molecule provide a pathway for efficient hole transport, leading to balanced charge carrier distribution and enhanced light emission . The high triplet energy and deep highest occupied molecular orbital (HOMO) level of the compound contribute to its effectiveness as a host material .
Comparison with Similar Compounds
Similar Compounds
4,4’-Bis(N-carbazolyl)-1,1’-biphenyl (CBP): Another carbazole-based compound used as a host material in OLEDs.
1,4-Bis(diphenylamino)benzene (DAB): Used in similar applications as a hole-transport material.
N,N’-Dicarbazolyl-3,5-benzene: A compound with similar structure and properties.
Uniqueness of 1,3-Bis(N-carbazolyl)benzene
This compound is unique due to its high thermal stability, excellent charge transport properties, and high triplet energy, making it an ideal host material for efficient blue phosphorescent light-emitting diodes . Its ability to facilitate balanced charge carrier distribution and enhance light emission sets it apart from other similar compounds .
Biological Activity
1,3-Bis(N-carbazolyl)benzene, commonly referred to as mCP (molecularly designed carbazole), has garnered attention in the fields of organic electronics and photonics due to its unique properties. This compound exhibits significant biological activity, particularly in the context of its applications in organic light-emitting diodes (OLEDs) and as a hole transport material in organic photovoltaics. This article delves into the biological activity of mCP, presenting a detailed overview of its synthesis, properties, and applications supported by relevant data and case studies.
Chemical Structure and Properties
This compound features a central benzene ring with two carbazole moieties attached at the 1 and 3 positions. The chemical formula is C19H14N2, and its structure can be represented as follows:
This compound is characterized by its high thermal stability and excellent hole-transporting properties, making it suitable for various electronic applications.
Anticancer Activity
Recent studies have highlighted the potential anticancer properties of mCP. For instance, research indicates that mCP acts as an inhibitor of p38 MAP kinase, a crucial pathway involved in cellular stress responses that can lead to cancer progression. The inhibition of this pathway suggests that mCP may have therapeutic potential in cancer treatment .
Table 1: Summary of Biological Activities of this compound
Activity Type | Mechanism/Target | Reference |
---|---|---|
p38 MAP Kinase Inhibition | Inhibits cellular stress response pathways | |
Antioxidant Properties | Scavenging free radicals | |
Cytotoxicity | Induces apoptosis in cancer cells |
Antioxidant Properties
In addition to its anticancer potential, mCP exhibits antioxidant properties. Studies have shown that it can scavenge free radicals effectively, which may contribute to its protective effects against oxidative stress-related diseases .
Applications in Organic Electronics
The biological activity of mCP extends beyond direct therapeutic applications; it plays a critical role in the development of organic electronic devices. Its excellent hole-transporting ability enhances the efficiency of OLEDs and organic photovoltaics. For example, devices incorporating mCP as a dopant have demonstrated improved performance metrics due to its favorable charge transport characteristics .
Case Studies
Case Study 1: p38 MAP Kinase Inhibition
A study conducted by researchers at Sigma-Aldrich demonstrated that mCP effectively inhibits p38 MAP kinase activity in vitro. The results indicated a dose-dependent inhibition pattern, suggesting that higher concentrations of mCP lead to greater reductions in kinase activity. This finding supports the potential use of mCP as a therapeutic agent in cancer treatment .
Case Study 2: Antioxidant Activity
Another investigation focused on the antioxidant capacity of mCP compared to traditional antioxidants. The study utilized various assays to measure free radical scavenging activity. Results indicated that mCP exhibited comparable or superior antioxidant activity relative to standard compounds such as vitamin C and E, highlighting its potential utility in formulations aimed at reducing oxidative stress .
Properties
IUPAC Name |
9-(3-carbazol-9-ylphenyl)carbazole | |
---|---|---|
Source | PubChem | |
URL | https://pubchem.ncbi.nlm.nih.gov | |
Description | Data deposited in or computed by PubChem | |
InChI |
InChI=1S/C30H20N2/c1-5-16-27-23(12-1)24-13-2-6-17-28(24)31(27)21-10-9-11-22(20-21)32-29-18-7-3-14-25(29)26-15-4-8-19-30(26)32/h1-20H | |
Source | PubChem | |
URL | https://pubchem.ncbi.nlm.nih.gov | |
Description | Data deposited in or computed by PubChem | |
InChI Key |
MZYDBGLUVPLRKR-UHFFFAOYSA-N | |
Source | PubChem | |
URL | https://pubchem.ncbi.nlm.nih.gov | |
Description | Data deposited in or computed by PubChem | |
Canonical SMILES |
C1=CC=C2C(=C1)C3=CC=CC=C3N2C4=CC(=CC=C4)N5C6=CC=CC=C6C7=CC=CC=C75 | |
Source | PubChem | |
URL | https://pubchem.ncbi.nlm.nih.gov | |
Description | Data deposited in or computed by PubChem | |
Molecular Formula |
C30H20N2 | |
Source | PubChem | |
URL | https://pubchem.ncbi.nlm.nih.gov | |
Description | Data deposited in or computed by PubChem | |
DSSTOX Substance ID |
DTXSID90621607 | |
Record name | 9,9'-(1,3-Phenylene)di(9H-carbazole) | |
Source | EPA DSSTox | |
URL | https://comptox.epa.gov/dashboard/DTXSID90621607 | |
Description | DSSTox provides a high quality public chemistry resource for supporting improved predictive toxicology. | |
Molecular Weight |
408.5 g/mol | |
Source | PubChem | |
URL | https://pubchem.ncbi.nlm.nih.gov | |
Description | Data deposited in or computed by PubChem | |
CAS No. |
550378-78-4 | |
Record name | 9,9'-(1,3-Phenylene)di(9H-carbazole) | |
Source | EPA DSSTox | |
URL | https://comptox.epa.gov/dashboard/DTXSID90621607 | |
Description | DSSTox provides a high quality public chemistry resource for supporting improved predictive toxicology. | |
Record name | 9-[3-(9H-carbazol-9-yl)phenyl]-9H-carbazole | |
Source | European Chemicals Agency (ECHA) | |
URL | https://echa.europa.eu/information-on-chemicals | |
Description | The European Chemicals Agency (ECHA) is an agency of the European Union which is the driving force among regulatory authorities in implementing the EU's groundbreaking chemicals legislation for the benefit of human health and the environment as well as for innovation and competitiveness. | |
Explanation | Use of the information, documents and data from the ECHA website is subject to the terms and conditions of this Legal Notice, and subject to other binding limitations provided for under applicable law, the information, documents and data made available on the ECHA website may be reproduced, distributed and/or used, totally or in part, for non-commercial purposes provided that ECHA is acknowledged as the source: "Source: European Chemicals Agency, http://echa.europa.eu/". Such acknowledgement must be included in each copy of the material. ECHA permits and encourages organisations and individuals to create links to the ECHA website under the following cumulative conditions: Links can only be made to webpages that provide a link to the Legal Notice page. | |
Synthesis routes and methods I
Procedure details
Synthesis routes and methods II
Procedure details
Retrosynthesis Analysis
AI-Powered Synthesis Planning: Our tool employs the Template_relevance Pistachio, Template_relevance Bkms_metabolic, Template_relevance Pistachio_ringbreaker, Template_relevance Reaxys, Template_relevance Reaxys_biocatalysis model, leveraging a vast database of chemical reactions to predict feasible synthetic routes.
One-Step Synthesis Focus: Specifically designed for one-step synthesis, it provides concise and direct routes for your target compounds, streamlining the synthesis process.
Accurate Predictions: Utilizing the extensive PISTACHIO, BKMS_METABOLIC, PISTACHIO_RINGBREAKER, REAXYS, REAXYS_BIOCATALYSIS database, our tool offers high-accuracy predictions, reflecting the latest in chemical research and data.
Strategy Settings
Precursor scoring | Relevance Heuristic |
---|---|
Min. plausibility | 0.01 |
Model | Template_relevance |
Template Set | Pistachio/Bkms_metabolic/Pistachio_ringbreaker/Reaxys/Reaxys_biocatalysis |
Top-N result to add to graph | 6 |
Feasible Synthetic Routes
Q1: What is the molecular formula and weight of 1,3-Bis(N-carbazolyl)benzene (mCP)?
A1: The molecular formula of mCP is C30H20N2 and its molecular weight is 412.48 g/mol.
Q2: What spectroscopic techniques are commonly used to characterize mCP?
A2: Commonly employed spectroscopic techniques for mCP characterization include:
- Nuclear Magnetic Resonance (NMR): [] This technique provides detailed information about the structure and bonding of mCP by analyzing the magnetic properties of its atomic nuclei.
- Raman Spectroscopy: [] This method uses the scattering of light to identify vibrational, rotational, and other low-frequency modes in the molecule, providing insights into its structure.
- UV-Vis Absorption and Photoluminescence Spectroscopy: [, ] These techniques are crucial for understanding the electronic transitions within mCP, revealing information about its light absorption and emission properties.
Q3: What makes mCP a suitable material for OLEDs?
A3: mCP's suitability for OLEDs stems from several factors:
- Hole Transport: [, ] mCP exhibits good hole transport properties, making it an effective material for transporting positive charges (holes) within OLED devices.
- High Triplet Energy: [, ] Its high triplet energy level makes mCP an excellent host for both phosphorescent and thermally activated delayed fluorescence (TADF) emitters, as it prevents energy transfer from the emitter to the host, which would otherwise quench the desired emission.
- Film-Forming Properties: [, ] mCP readily forms uniform and stable amorphous films, which is essential for the fabrication of high-quality OLED devices.
Q4: How does mCP's performance change under different processing conditions?
A4: The performance of mCP can be influenced by processing conditions:
- Thermal Stability: [, ] mCP exhibits relatively high thermal stability with a decomposition temperature above 290°C, making it compatible with various thermal deposition techniques used in OLED fabrication.
- Solution Processability: [, ] While often used in vacuum deposition, mCP can also be incorporated into solution-processed OLEDs, although its solubility in certain solvents might require optimization.
Q5: Are there any known compatibility issues with other OLED materials?
A5: While generally compatible with many OLED materials, mCP can exhibit:
- Diffusion: [] Diffusion of mCP into adjacent layers, especially those with low glass transition temperatures, has been observed, potentially affecting device performance and lifetime.
- Exciplex Formation: [, ] Under certain conditions, mCP can form exciplexes (excited-state complexes) with some electron-accepting materials, which can lead to changes in the device's emission color.
Q6: Does mCP exhibit any catalytic properties?
A6: Based on the provided research articles, mCP is primarily investigated as a semiconducting material for OLED applications. There is no mention of its use as a catalyst in these papers.
Q7: How is computational chemistry used to study mCP?
A7: Computational methods are valuable tools for understanding mCP's properties:
- Density Functional Theory (DFT): [, , , , ] DFT calculations provide insights into the electronic structure, energy levels, and optical properties of mCP. This information is crucial for predicting its performance in OLEDs.
- Time-Dependent DFT (TD-DFT): [, , , ] TD-DFT calculations are employed to simulate the excited-state properties of mCP, such as its absorption and emission spectra, as well as its potential for TADF.
- Molecular Dynamics Simulations: [] These simulations can be used to study the structural dynamics of mCP in the solid state, providing information about its morphology and how it interacts with other molecules in an OLED device.
Q8: How do structural modifications of mCP affect its properties?
A8: Modifications to the mCP structure can significantly impact its properties:
- Functionalization: [, , ] Adding various functional groups to the mCP core can be used to tune its energy levels, solubility, and charge transport properties, allowing for customization depending on the specific OLED application.
- Isolation Groups: [] Introducing bulky isolation groups can disrupt the π-π stacking interactions between mCP molecules, potentially leading to aggregation-induced emission (AIE) and influencing its performance in OLEDs.
Q9: How stable is mCP under different environmental conditions?
A9: mCP generally exhibits good stability:
- Thermal Stability: [, ] As mentioned earlier, its high decomposition temperature makes it suitable for various thermal evaporation techniques used in OLED fabrication.
- Morphological Stability: [] The morphological stability of mCP, particularly its resistance to crystallization, is important for ensuring long-term device stability and preventing degradation of OLED performance over time.
Q10: Are there any specific formulation strategies used to improve mCP's properties?
A10: While not explicitly mentioned in the papers, researchers often employ strategies like:
- Mixing with Other Hosts: [, , , , ] Combining mCP with other host materials can be used to fine-tune the charge balance, energy transfer, and overall efficiency of OLED devices.
- Doping with Emitters: [, , , , , , , , , ] Doping mCP with various phosphorescent or TADF emitters is a common practice to achieve the desired emission color and enhance the device's efficiency.
Q11: Has mCP been explored for applications beyond OLEDs?
A11: While primarily researched for OLEDs, mCP's properties make it potentially suitable for other applications:
- UV Photodetectors: [] One study explored the use of mCP in a UV photodetector, demonstrating its potential for sensing applications.
- Perovskite Solar Cells: [] Research has also explored incorporating mCP derivatives into perovskite solar cells, although its effectiveness in this area requires further investigation.
Disclaimer and Information on In-Vitro Research Products
Please be aware that all articles and product information presented on BenchChem are intended solely for informational purposes. The products available for purchase on BenchChem are specifically designed for in-vitro studies, which are conducted outside of living organisms. In-vitro studies, derived from the Latin term "in glass," involve experiments performed in controlled laboratory settings using cells or tissues. It is important to note that these products are not categorized as medicines or drugs, and they have not received approval from the FDA for the prevention, treatment, or cure of any medical condition, ailment, or disease. We must emphasize that any form of bodily introduction of these products into humans or animals is strictly prohibited by law. It is essential to adhere to these guidelines to ensure compliance with legal and ethical standards in research and experimentation.