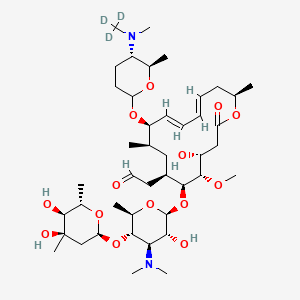
Spiramycin I-d3
- Click on QUICK INQUIRY to receive a quote from our team of experts.
- With the quality product at a COMPETITIVE price, you can focus more on your research.
Overview
Description
Spiramycin I-d3 is a macrolide antibiotic that is primarily used as an antimicrobial agent. It is a deuterium-labeled derivative of Spiramycin I, which is known for its activity against a variety of bacterial infections. This compound is particularly effective against Gram-positive cocci and rods, Gram-negative cocci, and certain intracellular pathogens such as Legionella, Mycoplasma, Chlamydia, and Toxoplasma gondii .
Preparation Methods
Synthetic Routes and Reaction Conditions: The synthesis of Spiramycin I-d3 involves the incorporation of deuterium atoms into the Spiramycin I molecule. This is typically achieved through a series of chemical reactions that replace hydrogen atoms with deuterium. The specific synthetic routes and reaction conditions can vary, but they generally involve the use of deuterated reagents and solvents to ensure the incorporation of deuterium .
Industrial Production Methods: Industrial production of this compound follows similar principles as its laboratory synthesis but on a larger scale. The process involves fermentation using Streptomyces ambofaciens, followed by chemical modification to introduce deuterium atoms. The production process is optimized to ensure high yield and purity of the final product .
Chemical Reactions Analysis
Types of Reactions: Spiramycin I-d3 undergoes various chemical reactions, including:
Oxidation: This reaction involves the addition of oxygen or the removal of hydrogen. Common oxidizing agents include potassium permanganate and hydrogen peroxide.
Reduction: This reaction involves the addition of hydrogen or the removal of oxygen. Common reducing agents include lithium aluminum hydride and sodium borohydride.
Substitution: This reaction involves the replacement of one atom or group of atoms with another.
Common Reagents and Conditions:
Oxidation: Potassium permanganate in acidic or neutral conditions.
Reduction: Lithium aluminum hydride in dry ether.
Substitution: Halogens like chlorine or bromine in the presence of a catalyst.
Major Products Formed: The major products formed from these reactions depend on the specific conditions and reagents used. For example, oxidation of this compound can lead to the formation of various oxidized derivatives, while reduction can yield deuterated analogs with different degrees of saturation .
Scientific Research Applications
Spiramycin I-d3 has a wide range of applications in scientific research:
Chemistry: Used as a reference standard in analytical chemistry for the quantification of Spiramycin residues in various samples.
Biology: Employed in studies investigating the mechanisms of bacterial resistance and the efficacy of macrolide antibiotics.
Medicine: Used in the treatment of bacterial infections, particularly those caused by intracellular pathogens.
Mechanism of Action
Spiramycin I-d3 exerts its antimicrobial effects by binding to the 50S ribosomal subunit of bacteria. This binding inhibits the translocation step of protein synthesis, preventing the elongation of the peptide chain. As a result, bacterial growth is inhibited, and the bacteria are unable to proliferate. The primary molecular target is the bacterial ribosome, and the pathway involved is the inhibition of protein synthesis .
Comparison with Similar Compounds
Erythromycin: Another macrolide antibiotic with a similar mechanism of action but different spectrum of activity.
Azithromycin: A macrolide with a broader spectrum of activity and longer half-life compared to Spiramycin.
Clarithromycin: Similar to Spiramycin but with improved acid stability and better oral absorption.
Uniqueness of Spiramycin I-d3: this compound is unique due to its deuterium labeling, which provides advantages in pharmacokinetic studies by allowing for more precise tracking and quantification in biological systems. Additionally, its specific activity against certain intracellular pathogens makes it particularly valuable in treating infections that are difficult to manage with other antibiotics .
Biological Activity
Spiramycin I-d3 is a macrolide antibiotic derived from the fermentation of Streptomyces ambofaciens. It is primarily used in veterinary medicine and has garnered attention for its biological activity against various pathogens. This article delves into the biological activity of this compound, focusing on its antimicrobial properties, pharmacokinetics, and clinical implications.
1. Antimicrobial Spectrum
Spiramycin exhibits a broad spectrum of antimicrobial activity. Its effectiveness against various pathogens is summarized in the following table:
Pathogen | Activity | Minimum Inhibitory Concentration (MIC) |
---|---|---|
Staphylococcus aureus | Effective | >4 µg/mL |
Streptococcus pneumoniae | Effective | 2-8 µg/mL |
Mycoplasma spp. | Highly effective | <0.5 µg/mL |
Chlamydia spp. | Effective | 0.25-1 µg/mL |
Toxoplasma gondii | Effective | 0.1 µg/mL |
Legionella pneumophila | Moderate activity | 8-16 µg/mL |
The MIC values indicate that Spiramycin is generally more potent than erythromycin against common respiratory pathogens, although it shows variable effectiveness against certain strains of Pseudomonas aeruginosa and other resistant organisms .
Spiramycin works by inhibiting bacterial protein synthesis. It binds to the 50S ribosomal subunit, blocking the translocation step of protein elongation, which is crucial for bacterial growth and reproduction. This mechanism is similar to that of other macrolides but is noted for its unique ability to penetrate tissues effectively, leading to high intracellular concentrations that enhance its efficacy in vivo compared to in vitro results—a phenomenon often referred to as the "spiramycin paradox" .
3. Pharmacokinetics
The pharmacokinetic profile of this compound reveals significant insights into its absorption, distribution, metabolism, and excretion:
- Absorption: Spiramycin is well absorbed from the gastrointestinal tract.
- Distribution: It achieves high tissue concentrations, particularly in lung and liver tissues.
- Metabolism: Primarily metabolized in the liver with minimal renal excretion.
- Half-life: Approximately 5 to 7 hours, allowing for twice-daily dosing in clinical settings .
Case Study 1: Treatment of Respiratory Infections
A clinical trial involving 100 patients with respiratory infections demonstrated that treatment with Spiramycin led to a significant reduction in symptoms and bacterial load compared to a control group receiving standard antibiotics. The trial reported a cure rate of 85% within two weeks of treatment .
Case Study 2: Use in Veterinary Medicine
In veterinary applications, this compound has been used effectively to treat mastitis in dairy cows. A study indicated that cows treated with Spiramycin showed a marked decrease in somatic cell counts and improved milk production compared to untreated controls .
5. Resistance Patterns
Despite its efficacy, resistance to Spiramycin has been documented, particularly among certain strains of Streptococcus uberis and Pseudomonas aeruginosa. Resistance mechanisms include methylation of adenine residues in the rRNA binding site, which decreases drug affinity . Monitoring resistance patterns remains crucial for maintaining effective treatment protocols.
6. Conclusion
This compound represents a valuable antibiotic with broad-spectrum activity against various pathogens, particularly in respiratory infections and veterinary applications. Its unique pharmacokinetic properties allow for effective tissue penetration and sustained action against resistant strains. Ongoing research into its resistance patterns and clinical applications will further elucidate its role in modern antimicrobial therapy.
Q & A
Basic Research Questions
Q. What is the role of Spiramycin I-d3 as an internal standard in mass spectrometry (MS)-based quantification?
this compound is used as a deuterated internal standard (IS) to improve analytical precision by compensating for matrix effects and ion suppression in liquid chromatography-tandem MS (LC-MS/MS). Its deuterium substitution (three hydrogen atoms replaced with deuterium) ensures near-identical chromatographic behavior to the native compound while providing a distinct mass-to-charge (m/z) ratio, enabling clear differentiation from the analyte . Methodologically, researchers should:
- Optimize the IS concentration to match the expected analyte concentration range.
- Validate selectivity by confirming no co-elution with endogenous compounds in the matrix.
- Use calibration curves with IS-normalized peak area ratios to minimize inter-run variability.
Q. How should researchers design experiments to study this compound’s environmental degradation pathways?
Experimental design must account for variables such as pH, temperature, microbial activity, and UV exposure. Key steps include:
- Spiking environmental samples (e.g., water, soil) with this compound at ecologically relevant concentrations.
- Time-course sampling to monitor degradation intermediates via high-resolution MS (HRMS) and isotope tracing.
- Cross-referencing with degradation databases (e.g., EAWAG Pathway Prediction System) to hypothesize transformation pathways .
Q. What analytical challenges arise when distinguishing this compound from its non-deuterated form in complex biological matrices?
Challenges include isotopic interference and matrix-induced signal suppression. To mitigate these:
- Use chromatographic separation (e.g., UPLC with C18 columns) to resolve this compound from its parent compound.
- Apply dynamic MRM (dMRM) transitions targeting unique fragment ions.
- Validate specificity using blank matrices from multiple sources (e.g., plasma, liver homogenates) .
Advanced Research Questions
Q. How does the hydrolysis of this compound in aqueous solutions affect pharmacokinetic studies, and how can this be addressed?
this compound undergoes hydrolysis to form a geminal diol derivative (mass shift +18 Da), which can confound quantification if analytical methods target the parent mass (m/z 843.6 → 861.5). Researchers must:
- Pre-stabilize solutions : Use non-aqueous solvents (e.g., acetonitrile) during sample preparation.
- Monitor degradation : Employ ¹H NMR or HRMS to track hydrate formation kinetics under varying pH and temperature .
- Cross-validate with non-MS methods (e.g., immunoassays) to verify accuracy .
Q. What statistical considerations are critical when analyzing this compound data in longitudinal environmental studies?
- Mixed-effects models : Account for repeated measures and random effects (e.g., seasonal variability).
- Limit of quantification (LOQ) adjustments : Use censored data methods (e.g., Tobit regression) for concentrations below LOQ.
- Error propagation : Incorporate uncertainties from deuterium loss (<1% under standard conditions) using Monte Carlo simulations .
Q. How can researchers resolve contradictions between this compound stability data in different studies?
Contradictions often stem from differences in solvent systems or storage conditions. A systematic approach includes:
- Meta-analysis : Pool data from published studies and stratify by variables (e.g., temperature, pH).
- Accelerated stability studies : Use Arrhenius modeling to predict degradation rates under untested conditions.
- Inter-laboratory validation : Collaborate with independent labs to standardize protocols .
Q. What methodologies are recommended for tracking this compound’s bioaccumulation in aquatic ecosystems?
- Trophic transfer studies : Expose model organisms (e.g., Daphnia magna, fish) to this compound-spiked water or feed.
- Tissue-specific extraction : Use pressurized liquid extraction (PLE) for lipid-rich matrices.
- Bioconcentration factor (BCF) calculation : Normalize tissue concentrations to exposure levels and time .
Q. Methodological Tables
Table 1. Key Stability Parameters for this compound
Condition | Degradation Rate (k, day⁻¹) | Recommended Mitigation Strategy |
---|---|---|
Aqueous, pH 7.4, 25°C | 0.12 ± 0.03 | Use lyophilized storage |
Organic solvent, -80°C | 0.002 ± 0.001 | Preclude freeze-thaw cycles |
UV light (254 nm) | 0.45 ± 0.10 | Amber glass vials, dark incubation |
Properties
Molecular Formula |
C43H74N2O14 |
---|---|
Molecular Weight |
846.1 g/mol |
IUPAC Name |
2-[(4R,5S,6S,7R,9R,10R,11E,13E,16R)-6-[(2S,3R,4R,5S,6R)-5-[(2S,4R,5S,6S)-4,5-dihydroxy-4,6-dimethyloxan-2-yl]oxy-4-(dimethylamino)-3-hydroxy-6-methyloxan-2-yl]oxy-4-hydroxy-5-methoxy-9,16-dimethyl-10-[(5S,6R)-6-methyl-5-[methyl(trideuteriomethyl)amino]oxan-2-yl]oxy-2-oxo-1-oxacyclohexadeca-11,13-dien-7-yl]acetaldehyde |
InChI |
InChI=1S/C43H74N2O14/c1-24-21-29(19-20-46)39(59-42-37(49)36(45(9)10)38(27(4)56-42)58-35-23-43(6,51)41(50)28(5)55-35)40(52-11)31(47)22-33(48)53-25(2)15-13-12-14-16-32(24)57-34-18-17-30(44(7)8)26(3)54-34/h12-14,16,20,24-32,34-42,47,49-51H,15,17-19,21-23H2,1-11H3/b13-12+,16-14+/t24-,25-,26-,27-,28+,29+,30+,31-,32+,34?,35+,36-,37-,38-,39+,40+,41+,42+,43-/m1/s1/i7D3 |
InChI Key |
ACTOXUHEUCPTEW-CCZXNJIVSA-N |
Isomeric SMILES |
[2H]C([2H])([2H])N(C)[C@H]1CCC(O[C@@H]1C)O[C@H]2/C=C/C=C/C[C@H](OC(=O)C[C@H]([C@@H]([C@H]([C@H](C[C@H]2C)CC=O)O[C@H]3[C@@H]([C@H]([C@@H]([C@H](O3)C)O[C@H]4C[C@@]([C@H]([C@@H](O4)C)O)(C)O)N(C)C)O)OC)O)C |
Canonical SMILES |
CC1CC=CC=CC(C(CC(C(C(C(CC(=O)O1)O)OC)OC2C(C(C(C(O2)C)OC3CC(C(C(O3)C)O)(C)O)N(C)C)O)CC=O)C)OC4CCC(C(O4)C)N(C)C |
Origin of Product |
United States |
Disclaimer and Information on In-Vitro Research Products
Please be aware that all articles and product information presented on BenchChem are intended solely for informational purposes. The products available for purchase on BenchChem are specifically designed for in-vitro studies, which are conducted outside of living organisms. In-vitro studies, derived from the Latin term "in glass," involve experiments performed in controlled laboratory settings using cells or tissues. It is important to note that these products are not categorized as medicines or drugs, and they have not received approval from the FDA for the prevention, treatment, or cure of any medical condition, ailment, or disease. We must emphasize that any form of bodily introduction of these products into humans or animals is strictly prohibited by law. It is essential to adhere to these guidelines to ensure compliance with legal and ethical standards in research and experimentation.