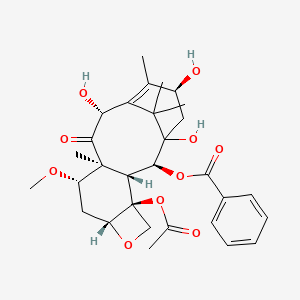
10-Deacetyl-7-methyl Baccatin III
- Click on QUICK INQUIRY to receive a quote from our team of experts.
- With the quality product at a COMPETITIVE price, you can focus more on your research.
Overview
Description
10-Deacetyl-7-methyl Baccatin III is a natural organic compound isolated from the yew tree (Taxus species). It is a crucial precursor in the biosynthesis of the anti-cancer drug docetaxel (Taxotere) and paclitaxel (Taxol) .
Preparation Methods
Synthetic Routes and Reaction Conditions: 10-Deacetyl-7-methyl Baccatin III can be synthesized through various methods. One common approach involves the extraction from the needles of Taxus baccata L. using solvents like ethanol and methanol . The extraction process is optimized using response surface methodology to determine the effects of temperature, extraction time, and particle size . Another method involves the chemical synthesis using 9-DHB (13-acetyl-9-dihydrobaccatin) III as a substrate . This process includes steps such as adding 9-DHB powder and methanol into a reaction kettle, heating, adding hydrazine hydrate, and performing chromatography to obtain the final product .
Industrial Production Methods: Industrial production of this compound often involves the use of renewable Taxus needles and in situ whole-cell catalysis . This method integrates the extraction of 10-DAB from Taxus needles and whole-cell biotransformation to produce baccatin III . The process is optimized to achieve high yields and efficient production .
Chemical Reactions Analysis
10-Deacetyl-7-methyl Baccatin III undergoes various chemical reactions, including oxidation, reduction, and substitution . Common reagents used in these reactions include acetyl-CoA and hydrazine hydrate . One significant reaction is the conversion of 10-Deacetylbaccatin III to baccatin III using 10-deacetylbaccatin III 10-O-acetyltransferase . The major products formed from these reactions include baccatin III and other derivatives used in the synthesis of anti-cancer drugs .
Scientific Research Applications
10-Deacetyl-7-methyl Baccatin III has numerous scientific research applications. It is primarily used as a precursor in the semi-synthesis of paclitaxel (Taxol) and docetaxel (Taxotere), which are widely used in cancer chemotherapy . The compound is also used to study the taxol biosynthetic pathway and the effects of various reagents and conditions on its synthesis . Additionally, it has applications in the pharmaceutical industry for the development of new anti-cancer drugs and in biological research to understand its mechanism of action .
Mechanism of Action
The mechanism of action of 10-Deacetyl-7-methyl Baccatin III involves its conversion to paclitaxel (Taxol) and docetaxel (Taxotere), which exert their effects by promoting abnormal polymerization of tubulin and disrupting mitosis . This leads to the inhibition of cell division and the induction of apoptosis in cancer cells . The molecular targets include tubulin and microtubules, which are essential for cell division .
Comparison with Similar Compounds
10-Deacetyl-7-methyl Baccatin III is similar to other compounds in the 10-Deacetylbaccatin series, such as 10-Deacetylbaccatin V, 10-Deacetylbaccatin VI, and 13-Epi-10-Deacetylbaccatin III . These compounds share similar structures and are also precursors to anti-cancer drugs . this compound is unique in its specific role as a precursor to both paclitaxel and docetaxel, making it a valuable compound in cancer research and treatment .
Properties
Molecular Formula |
C30H38O10 |
---|---|
Molecular Weight |
558.6 g/mol |
IUPAC Name |
[(2S,3R,4S,7R,9S,10S,12R,15S)-4-acetyloxy-1,12,15-trihydroxy-9-methoxy-10,14,17,17-tetramethyl-11-oxo-6-oxatetracyclo[11.3.1.03,10.04,7]heptadec-13-en-2-yl] benzoate |
InChI |
InChI=1S/C30H38O10/c1-15-18(32)13-30(36)25(39-26(35)17-10-8-7-9-11-17)23-28(5,24(34)22(33)21(15)27(30,3)4)19(37-6)12-20-29(23,14-38-20)40-16(2)31/h7-11,18-20,22-23,25,32-33,36H,12-14H2,1-6H3/t18-,19-,20+,22+,23-,25-,28+,29-,30?/m0/s1 |
InChI Key |
IWJSBKNWKLQICP-ARYQKKAUSA-N |
Isomeric SMILES |
CC1=C2[C@H](C(=O)[C@@]3([C@H](C[C@@H]4[C@]([C@H]3[C@@H](C(C2(C)C)(C[C@@H]1O)O)OC(=O)C5=CC=CC=C5)(CO4)OC(=O)C)OC)C)O |
Canonical SMILES |
CC1=C2C(C(=O)C3(C(CC4C(C3C(C(C2(C)C)(CC1O)O)OC(=O)C5=CC=CC=C5)(CO4)OC(=O)C)OC)C)O |
Origin of Product |
United States |
Disclaimer and Information on In-Vitro Research Products
Please be aware that all articles and product information presented on BenchChem are intended solely for informational purposes. The products available for purchase on BenchChem are specifically designed for in-vitro studies, which are conducted outside of living organisms. In-vitro studies, derived from the Latin term "in glass," involve experiments performed in controlled laboratory settings using cells or tissues. It is important to note that these products are not categorized as medicines or drugs, and they have not received approval from the FDA for the prevention, treatment, or cure of any medical condition, ailment, or disease. We must emphasize that any form of bodily introduction of these products into humans or animals is strictly prohibited by law. It is essential to adhere to these guidelines to ensure compliance with legal and ethical standards in research and experimentation.