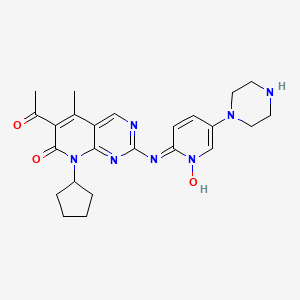
Palbociclib Pyridine N-Oxide
- Click on QUICK INQUIRY to receive a quote from our team of experts.
- With the quality product at a COMPETITIVE price, you can focus more on your research.
Overview
Description
Palbociclib Pyridine N-Oxide: is a derivative of Palbociclib, a well-known cyclin-dependent kinase inhibitor used primarily in the treatment of hormone receptor-positive, HER2-negative advanced or metastatic breast cancer . The compound is characterized by the presence of a pyridine N-oxide moiety, which can influence its chemical properties and biological activities .
Preparation Methods
Synthetic Routes and Reaction Conditions: The synthesis of Palbociclib Pyridine N-Oxide typically involves multiple steps, starting from readily available raw materials. One reported method involves the following steps :
Nucleophilic Substitution: Starting with 2-(methylthio)pyrimidin-4-(3H)-one, a nucleophilic substitution reaction is carried out using thionyl chloride.
Bromination: The intermediate is then brominated.
Cyclopentylamine Substitution: This is followed by a nucleophilic substitution with cyclopentylamine.
Heck Reaction and Ring Closure: A one-pot two-step method involving the Heck reaction and ring closure sequence.
Oxidation and Bromination: The intermediate undergoes oxidation and further bromination.
Cross-Coupling Reaction: A cross-coupling reaction is performed to introduce the pyridine N-oxide moiety.
Final Steps: The final steps involve aqueous workup to yield this compound.
Industrial Production Methods: The industrial production of this compound follows similar synthetic routes but is optimized for higher yields and scalability. The process involves the use of inexpensive raw materials and reagents, readily controllable reaction conditions, and environmentally friendly practices .
Chemical Reactions Analysis
Types of Reactions: Palbociclib Pyridine N-Oxide undergoes various chemical reactions, including:
Oxidation: The pyridine moiety can be oxidized using peracids such as peracetic acid or perbenzoic acid.
Reduction: The compound can be reduced under specific conditions to yield different derivatives.
Substitution: Nucleophilic and electrophilic substitution reactions are common, especially involving the pyridine ring.
Common Reagents and Conditions:
Oxidation: Peracids, urea-hydrogen peroxide complex, sodium perborate, and methylrhenium trioxide as catalysts.
Reduction: Various reducing agents depending on the desired product.
Substitution: Thionyl chloride, cyclopentylamine, and other nucleophiles or electrophiles.
Major Products: The major products formed from these reactions include various derivatives of this compound, which can be further studied for their biological activities and potential therapeutic applications.
Scientific Research Applications
Chemistry: Palbociclib Pyridine N-Oxide is used in the synthesis of novel cyclin-dependent kinase inhibitors. Its unique structure allows for the exploration of structure-activity relationships and the development of more selective and potent inhibitors .
Biology and Medicine: The compound is studied for its potential in cancer therapy, particularly in targeting cyclin-dependent kinases 4 and 6. It has shown promise in inducing cell cycle arrest and inhibiting tumor growth .
Industry: In the pharmaceutical industry, this compound serves as a reference standard and is used in the development of new therapeutic agents .
Mechanism of Action
Palbociclib Pyridine N-Oxide exerts its effects by inhibiting cyclin-dependent kinases 4 and 6 (CDK4/6). These kinases are crucial for the progression of the cell cycle from the G1 phase to the S phase. By inhibiting CDK4/6, the compound prevents the phosphorylation of the retinoblastoma protein, thereby blocking cell cycle progression and inducing cell cycle arrest .
Comparison with Similar Compounds
Ribociclib: Another CDK4/6 inhibitor with a similar mechanism of action.
Abemaciclib: A CDK4/6 inhibitor with a slightly different chemical structure and pharmacokinetic profile.
Uniqueness: Palbociclib Pyridine N-Oxide is unique due to the presence of the pyridine N-oxide moiety, which can enhance its selectivity and potency as a CDK4/6 inhibitor. This structural modification allows for improved pharmacological properties and potential therapeutic benefits .
Properties
CAS No. |
2098673-40-4 |
---|---|
Molecular Formula |
C24H29N7O3 |
Molecular Weight |
463.5 g/mol |
IUPAC Name |
6-acetyl-8-cyclopentyl-2-[(E)-(1-hydroxy-5-piperazin-1-ylpyridin-2-ylidene)amino]-5-methylpyrido[2,3-d]pyrimidin-7-one |
InChI |
InChI=1S/C24H29N7O3/c1-15-19-13-26-24(27-20-8-7-18(14-30(20)34)29-11-9-25-10-12-29)28-22(19)31(17-5-3-4-6-17)23(33)21(15)16(2)32/h7-8,13-14,17,25,34H,3-6,9-12H2,1-2H3/b27-20+ |
InChI Key |
FDCZOGMXUYLOLB-NHFJDJAPSA-N |
Isomeric SMILES |
CC1=C(C(=O)N(C2=NC(=NC=C12)/N=C/3\C=CC(=CN3O)N4CCNCC4)C5CCCC5)C(=O)C |
Canonical SMILES |
CC1=C(C(=O)N(C2=NC(=NC=C12)N=C3C=CC(=CN3O)N4CCNCC4)C5CCCC5)C(=O)C |
Origin of Product |
United States |
Disclaimer and Information on In-Vitro Research Products
Please be aware that all articles and product information presented on BenchChem are intended solely for informational purposes. The products available for purchase on BenchChem are specifically designed for in-vitro studies, which are conducted outside of living organisms. In-vitro studies, derived from the Latin term "in glass," involve experiments performed in controlled laboratory settings using cells or tissues. It is important to note that these products are not categorized as medicines or drugs, and they have not received approval from the FDA for the prevention, treatment, or cure of any medical condition, ailment, or disease. We must emphasize that any form of bodily introduction of these products into humans or animals is strictly prohibited by law. It is essential to adhere to these guidelines to ensure compliance with legal and ethical standards in research and experimentation.