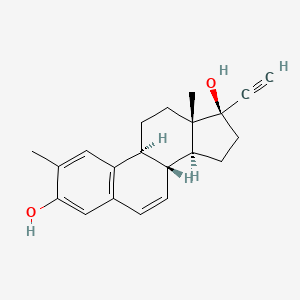
Ethynylestradiol Impurity M
- Click on QUICK INQUIRY to receive a quote from our team of experts.
- With the quality product at a COMPETITIVE price, you can focus more on your research.
Overview
Description
Ethynylestradiol Impurity M is a chemical compound that is often encountered as an impurity in the synthesis and production of Ethynylestradiol, a synthetic estrogen used in various pharmaceutical applications, particularly in oral contraceptives and hormone replacement therapy . This impurity is significant in pharmaceutical research and quality control to ensure the safety and efficacy of Ethynylestradiol-containing products .
Preparation Methods
Synthetic Routes and Reaction Conditions: The preparation of Ethynylestradiol Impurity M involves complex organic synthesis techniques. The synthetic routes typically include the use of various solvents such as ethanol, acetone, dichloromethane, and chloroform . The reaction conditions often require precise control of temperature, pH, and reaction time to ensure the formation of the desired impurity.
Industrial Production Methods: In industrial settings, the production of this compound is closely monitored to minimize its presence in the final product. Advanced chromatographic techniques and rigorous quality control measures are employed to detect and quantify this impurity .
Chemical Reactions Analysis
Types of Reactions: Ethynylestradiol Impurity M undergoes various chemical reactions, including oxidation, reduction, and substitution reactions . These reactions are crucial for understanding the stability and reactivity of the compound.
Common Reagents and Conditions: Common reagents used in these reactions include oxidizing agents like potassium permanganate and reducing agents such as sodium borohydride . The reaction conditions often involve controlled temperatures and specific pH levels to achieve the desired transformations.
Major Products Formed: The major products formed from these reactions depend on the specific reagents and conditions used. For example, oxidation reactions may yield hydroxylated derivatives, while reduction reactions can produce dehydrogenated compounds .
Scientific Research Applications
Ethynylestradiol Impurity M has several scientific research applications, including:
Mechanism of Action
The mechanism of action of Ethynylestradiol Impurity M is closely related to that of Ethynylestradiol. It interacts with estrogen receptors in the body, influencing various physiological processes . The molecular targets include estrogen receptors in reproductive tissues, the liver, and the hypothalamus . The pathways involved in its action include the modulation of gene expression and the regulation of hormonal balance .
Comparison with Similar Compounds
Ethynylestradiol Impurity M can be compared with other similar compounds, such as:
- Ethynylestradiol Impurity A
- Ethynylestradiol Impurity B
- Ethynylestradiol Impurity C
- Ethynylestradiol Impurity D
Each of these impurities has unique chemical properties and biological effects. This compound is unique in its specific structural characteristics and its formation during the synthesis of Ethynylestradiol .
Biological Activity
Ethynylestradiol (EE) is a synthetic estrogen widely used in hormonal contraceptives and hormone replacement therapy. Its impurities, particularly Ethynylestradiol Impurity M, have garnered attention due to their potential biological activities and implications for human health. This article explores the biological activity of this compound, including its effects on reproductive health, metabolic pathways, and associated risks.
Overview of this compound
This compound is a byproduct formed during the synthesis of EE. While the primary compound is well-studied, the biological implications of its impurities are less understood. Recent studies have begun to elucidate the effects of these impurities on various biological systems.
Biological Activity
1. Estrogenic Activity:
Ethynylestradiol and its impurities exhibit estrogenic activity by binding to estrogen receptors (ERs), which can lead to various physiological effects. Research indicates that EE can disrupt endocrine functions in aquatic organisms, such as fish, affecting reproductive endpoints like hormone levels and gonadal morphology .
2. Metabolic Pathways:
EE undergoes extensive metabolism primarily via cytochrome P450 enzymes, leading to the formation of several metabolites, including impurities. The presence of this compound may influence the metabolism of EE and other co-administered drugs by inhibiting metabolic enzymes such as CYP1A2 and CYP3A4 . This inhibition can result in increased plasma concentrations of drugs metabolized by these enzymes, raising concerns about potential drug interactions.
Case Study 1: Impact on Fish Reproductive Health
A study examined the effects of EE on the protandrous gilthead seabream. Fish exposed to EE at concentrations of 5 µg/g food exhibited significant alterations in reproductive hormones, gonad morphology, and gene expression related to steroidogenesis. Notably, recovery from these effects was observed over time post-exposure, indicating potential long-term impacts on fish populations .
Case Study 2: Clinical Implications in Humans
In a clinical setting, the use of COCs containing EE has been associated with adverse health outcomes, including an increased risk of venous thromboembolism (VTE). A study highlighted that users of COCs containing drospirenone and EE exhibited altered phase I/II biotransformation activities, potentially leading to increased cardiovascular risks due to lipid peroxidation . The presence of impurities like this compound may exacerbate these risks by further inhibiting metabolic pathways.
Table 1: Summary of Biological Effects
Properties
Molecular Formula |
C21H24O2 |
---|---|
Molecular Weight |
308.4 g/mol |
IUPAC Name |
(8R,9S,13S,14S,17R)-17-ethynyl-2,13-dimethyl-9,11,12,14,15,16-hexahydro-8H-cyclopenta[a]phenanthrene-3,17-diol |
InChI |
InChI=1S/C21H24O2/c1-4-21(23)10-8-18-16-6-5-14-12-19(22)13(2)11-17(14)15(16)7-9-20(18,21)3/h1,5-6,11-12,15-16,18,22-23H,7-10H2,2-3H3/t15-,16+,18-,20-,21-/m0/s1 |
InChI Key |
WZHDKGOPJSUDCC-OKSLILNBSA-N |
Isomeric SMILES |
CC1=CC2=C(C=C[C@@H]3[C@@H]2CC[C@]4([C@H]3CC[C@]4(C#C)O)C)C=C1O |
Canonical SMILES |
CC1=CC2=C(C=CC3C2CCC4(C3CCC4(C#C)O)C)C=C1O |
Origin of Product |
United States |
Disclaimer and Information on In-Vitro Research Products
Please be aware that all articles and product information presented on BenchChem are intended solely for informational purposes. The products available for purchase on BenchChem are specifically designed for in-vitro studies, which are conducted outside of living organisms. In-vitro studies, derived from the Latin term "in glass," involve experiments performed in controlled laboratory settings using cells or tissues. It is important to note that these products are not categorized as medicines or drugs, and they have not received approval from the FDA for the prevention, treatment, or cure of any medical condition, ailment, or disease. We must emphasize that any form of bodily introduction of these products into humans or animals is strictly prohibited by law. It is essential to adhere to these guidelines to ensure compliance with legal and ethical standards in research and experimentation.