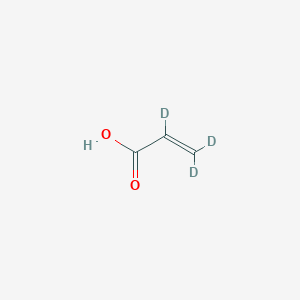
Acrylic Acid-d3
- Click on QUICK INQUIRY to receive a quote from our team of experts.
- With the quality product at a COMPETITIVE price, you can focus more on your research.
Overview
Description
It is a colorless liquid with the molecular formula C3HD3O2 and a molecular weight of 75.08 g/mol . This compound is used as a stable isotope-labeled compound in various scientific research applications.
Preparation Methods
Synthetic Routes and Reaction Conditions
Acrylic Acid-d3 can be synthesized through the deuteration of acrylic acid. The process involves the exchange of hydrogen atoms with deuterium atoms. One common method is the catalytic exchange reaction using deuterium gas (D2) in the presence of a catalyst such as palladium on carbon (Pd/C). The reaction is typically carried out under mild conditions to ensure complete deuteration .
Industrial Production Methods
Industrial production of this compound follows similar principles but on a larger scale. The process involves the use of deuterium oxide (D2O) as a deuterium source and a suitable catalyst to facilitate the exchange reaction. The reaction conditions are optimized to achieve high yields and purity of the deuterated product .
Chemical Reactions Analysis
Types of Reactions
Acrylic Acid-d3 undergoes various chemical reactions characteristic of unsaturated carboxylic acids. These include:
Addition Reactions: The double bond in this compound can participate in addition reactions with hydrogen, halogens, and other nucleophiles.
Polymerization: This compound readily undergoes polymerization to form polyacrylic acid or copolymers with other monomers.
Esterification: The carboxyl group can react with alcohols to form esters.
Common Reagents and Conditions
Hydrogenation: Using hydrogen gas (H2) and a palladium catalyst.
Halogenation: Using halogens like chlorine (Cl2) or bromine (Br2).
Polymerization: Initiated by heat, light, or peroxides.
Esterification: Using alcohols and acid catalysts.
Major Products Formed
Polyacrylic Acid: Formed through polymerization.
Acrylic Esters: Formed through esterification with various alcohols.
Scientific Research Applications
Acrylic Acid-d3 is widely used in scientific research due to its stable isotope labeling. Some applications include:
Chemistry: Used in studies involving reaction mechanisms and kinetics.
Biology: Utilized in metabolic studies and tracing biochemical pathways.
Medicine: Employed in drug development and pharmacokinetic studies.
Industry: Used in the production of specialty polymers and materials .
Mechanism of Action
The mechanism of action of Acrylic Acid-d3 involves its participation in chemical reactions as a labeled compound. The deuterium atoms in this compound provide a distinct mass difference, allowing researchers to trace the compound through various reactions and pathways. This helps in understanding reaction mechanisms, studying metabolic processes, and developing new materials .
Comparison with Similar Compounds
Similar Compounds
Acrylic Acid: The non-deuterated form with similar chemical properties but without the isotope labeling.
Methacrylic Acid: Another unsaturated carboxylic acid with a methyl group on the alpha carbon.
Crotonic Acid: An unsaturated carboxylic acid with a trans double bond.
Uniqueness
Acrylic Acid-d3 is unique due to its deuterium labeling, which makes it valuable for research applications that require tracing and studying reaction mechanisms. The presence of deuterium atoms provides a distinct advantage in nuclear magnetic resonance (NMR) spectroscopy and mass spectrometry, allowing for precise analysis and identification of reaction intermediates and products .
Properties
Molecular Formula |
C3H4O2 |
---|---|
Molecular Weight |
75.08 g/mol |
IUPAC Name |
2,3,3-trideuterioprop-2-enoic acid |
InChI |
InChI=1S/C3H4O2/c1-2-3(4)5/h2H,1H2,(H,4,5)/i1D2,2D |
InChI Key |
NIXOWILDQLNWCW-FUDHJZNOSA-N |
Isomeric SMILES |
[2H]C(=C([2H])C(=O)O)[2H] |
Canonical SMILES |
C=CC(=O)O |
Origin of Product |
United States |
Disclaimer and Information on In-Vitro Research Products
Please be aware that all articles and product information presented on BenchChem are intended solely for informational purposes. The products available for purchase on BenchChem are specifically designed for in-vitro studies, which are conducted outside of living organisms. In-vitro studies, derived from the Latin term "in glass," involve experiments performed in controlled laboratory settings using cells or tissues. It is important to note that these products are not categorized as medicines or drugs, and they have not received approval from the FDA for the prevention, treatment, or cure of any medical condition, ailment, or disease. We must emphasize that any form of bodily introduction of these products into humans or animals is strictly prohibited by law. It is essential to adhere to these guidelines to ensure compliance with legal and ethical standards in research and experimentation.