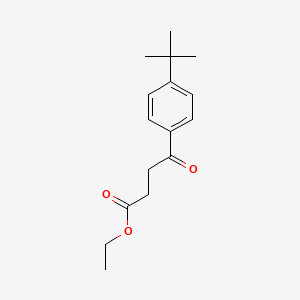
Ethyl 4-(4-T-butylphenyl)-4-oxobutyrate
Overview
Description
Ethyl 4-(4-T-butylphenyl)-4-oxobutyrate is an organic compound that belongs to the class of esters It is characterized by the presence of a tert-butyl group attached to a phenyl ring, which is further connected to a butyrate ester
Preparation Methods
Synthetic Routes and Reaction Conditions
The synthesis of Ethyl 4-(4-T-butylphenyl)-4-oxobutyrate typically involves the esterification of 4-(4-T-butylphenyl)-4-oxobutyric acid with ethanol. The reaction is usually catalyzed by an acid such as sulfuric acid or hydrochloric acid. The process involves heating the reactants under reflux conditions to facilitate the esterification reaction.
Industrial Production Methods
In an industrial setting, the production of this compound can be scaled up using continuous flow reactors. These reactors allow for precise control over reaction conditions, such as temperature and pressure, ensuring high yields and purity of the final product. The use of catalysts and solvents is optimized to minimize waste and improve efficiency.
Chemical Reactions Analysis
Types of Reactions
Ethyl 4-(4-T-butylphenyl)-4-oxobutyrate can undergo various chemical reactions, including:
Oxidation: The compound can be oxidized to form corresponding carboxylic acids.
Reduction: Reduction reactions can convert the ketone group to an alcohol.
Substitution: The ester group can participate in nucleophilic substitution reactions.
Common Reagents and Conditions
Oxidation: Common oxidizing agents include potassium permanganate and chromium trioxide.
Reduction: Reducing agents such as lithium aluminum hydride or sodium borohydride are typically used.
Substitution: Nucleophiles like hydroxide ions or amines can be used under basic conditions.
Major Products Formed
Oxidation: 4-(4-T-butylphenyl)-4-oxobutyric acid.
Reduction: Ethyl 4-(4-T-butylphenyl)-4-hydroxybutyrate.
Substitution: Various substituted esters depending on the nucleophile used.
Scientific Research Applications
Ethyl 4-(4-T-butylphenyl)-4-oxobutyrate has several applications in scientific research:
Chemistry: It is used as an intermediate in the synthesis of more complex organic molecules.
Biology: The compound can be used in studies involving enzyme-catalyzed reactions and metabolic pathways.
Industry: Used in the production of polymers and resins due to its ester functionality.
Mechanism of Action
The mechanism of action of Ethyl 4-(4-T-butylphenyl)-4-oxobutyrate involves its interaction with various molecular targets. The ester group can undergo hydrolysis to release the corresponding acid and alcohol, which can then participate in further biochemical reactions. The tert-butyl group provides steric hindrance, affecting the compound’s reactivity and interaction with enzymes and other proteins.
Comparison with Similar Compounds
Ethyl 4-(4-T-butylphenyl)-4-oxobutyrate can be compared with similar compounds such as:
Ethyl 4-phenyl-4-oxobutyrate: Lacks the tert-butyl group, resulting in different steric and electronic properties.
Mthis compound: Similar structure but with a methyl ester instead of an ethyl ester, affecting its reactivity and solubility.
Ethyl 4-(4-methylphenyl)-4-oxobutyrate: The presence of a methyl group instead of a tert-butyl group alters its steric effects and reactivity.
Biological Activity
Ethyl 4-(4-T-butylphenyl)-4-oxobutyrate, a compound with the molecular formula CHO and a molecular weight of approximately 262.35 g/mol, has garnered attention for its potential biological activities, particularly in the realms of anti-inflammatory and anti-cancer properties. This article delves into the biological activity of this compound, synthesizing findings from various studies and providing insights into its mechanisms of action.
Chemical Structure and Properties
The compound is characterized by a tert-butyl group attached to a phenyl ring and an oxobutyrate moiety , which contribute to its unique reactivity and biological activity. The structural features enable it to interact with various enzymes, influencing their activity and potentially leading to therapeutic applications.
Anti-Cancer Properties
One of the most significant areas of interest for this compound is its anti-cancer activity . Studies suggest that compounds with similar structures can inhibit ferrochelatase (FECH), an enzyme critical in heme biosynthesis. Inhibition of FECH leads to an accumulation of protoporphyrin IX (PPIX), which has been linked to anti-angiogenic effects—an important factor in cancer progression.
A notable study demonstrated that certain derivatives effectively blocked tube formation in human retinal endothelial cells (HRECs), indicating their potential as anti-angiogenic agents. The inhibition of FECH was confirmed through enzymatic assays and cellular studies, suggesting that this compound could play a role in cancer therapy by targeting angiogenesis .
The exact mechanism of action for this compound remains largely unexplored due to the limited research available. However, its structural characteristics suggest it may bind to specific enzymes, altering their activity. For instance, the interaction with FECH could lead to decreased heme production, which is essential for various cellular functions and may result in reduced tumor growth .
Synthesis and Derivatives
The synthesis of this compound involves several methods, including reactions with tert-butyl 2-oxobutanoate. Various derivatives have been synthesized and evaluated for their biological activities, revealing that modifications in structure can significantly impact potency against cancer cell lines .
Comparative Analysis with Similar Compounds
To better understand the significance of this compound, a comparison with structurally similar compounds is useful:
Compound Name | Structure Similarity | Unique Features |
---|---|---|
Ethyl 3-oxobutanoate | Ketone group | Widely used in organic synthesis |
Ethyl 2-oxo-4-phenylbutanoate | Phenyl group | Exhibits different reactivity patterns |
Ethyl 3-(4-tert-butylphenyl)propanoate | Propanoate moiety | Different alkane chain length affecting properties |
This compound stands out due to its specific combination of functional groups that impart distinct reactivity and biological activity compared to other similar compounds .
Properties
IUPAC Name |
ethyl 4-(4-tert-butylphenyl)-4-oxobutanoate | |
---|---|---|
Source | PubChem | |
URL | https://pubchem.ncbi.nlm.nih.gov | |
Description | Data deposited in or computed by PubChem | |
InChI |
InChI=1S/C16H22O3/c1-5-19-15(18)11-10-14(17)12-6-8-13(9-7-12)16(2,3)4/h6-9H,5,10-11H2,1-4H3 | |
Source | PubChem | |
URL | https://pubchem.ncbi.nlm.nih.gov | |
Description | Data deposited in or computed by PubChem | |
InChI Key |
PXHOSTSMXOPDRQ-UHFFFAOYSA-N | |
Source | PubChem | |
URL | https://pubchem.ncbi.nlm.nih.gov | |
Description | Data deposited in or computed by PubChem | |
Canonical SMILES |
CCOC(=O)CCC(=O)C1=CC=C(C=C1)C(C)(C)C | |
Source | PubChem | |
URL | https://pubchem.ncbi.nlm.nih.gov | |
Description | Data deposited in or computed by PubChem | |
Molecular Formula |
C16H22O3 | |
Source | PubChem | |
URL | https://pubchem.ncbi.nlm.nih.gov | |
Description | Data deposited in or computed by PubChem | |
DSSTOX Substance ID |
DTXSID60599304 | |
Record name | Ethyl 4-(4-tert-butylphenyl)-4-oxobutanoate | |
Source | EPA DSSTox | |
URL | https://comptox.epa.gov/dashboard/DTXSID60599304 | |
Description | DSSTox provides a high quality public chemistry resource for supporting improved predictive toxicology. | |
Molecular Weight |
262.34 g/mol | |
Source | PubChem | |
URL | https://pubchem.ncbi.nlm.nih.gov | |
Description | Data deposited in or computed by PubChem | |
CAS No. |
75237-09-1 | |
Record name | Ethyl 4-(4-tert-butylphenyl)-4-oxobutanoate | |
Source | EPA DSSTox | |
URL | https://comptox.epa.gov/dashboard/DTXSID60599304 | |
Description | DSSTox provides a high quality public chemistry resource for supporting improved predictive toxicology. | |
Synthesis routes and methods
Procedure details
Disclaimer and Information on In-Vitro Research Products
Please be aware that all articles and product information presented on BenchChem are intended solely for informational purposes. The products available for purchase on BenchChem are specifically designed for in-vitro studies, which are conducted outside of living organisms. In-vitro studies, derived from the Latin term "in glass," involve experiments performed in controlled laboratory settings using cells or tissues. It is important to note that these products are not categorized as medicines or drugs, and they have not received approval from the FDA for the prevention, treatment, or cure of any medical condition, ailment, or disease. We must emphasize that any form of bodily introduction of these products into humans or animals is strictly prohibited by law. It is essential to adhere to these guidelines to ensure compliance with legal and ethical standards in research and experimentation.