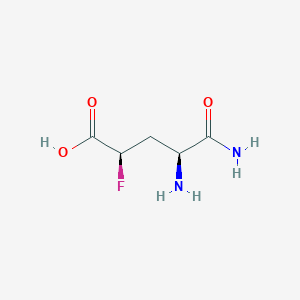
(2R,4S)-4,5-diamino-2-fluoro-5-oxopentanoic acid
- Click on QUICK INQUIRY to receive a quote from our team of experts.
- With the quality product at a COMPETITIVE price, you can focus more on your research.
Overview
Description
(2R,4S)-4,5-diamino-2-fluoro-5-oxopentanoic acid is a synthetic organic compound with potential applications in various fields of chemistry, biology, and medicine. This compound is characterized by the presence of amino, fluoro, and oxo functional groups, which contribute to its unique chemical properties and reactivity.
Preparation Methods
Synthetic Routes and Reaction Conditions
The synthesis of (2R,4S)-4,5-diamino-2-fluoro-5-oxopentanoic acid typically involves multi-step organic reactions. One common approach is the diastereoselective synthesis, which ensures the correct stereochemistry of the compound. The synthesis may start with commercially available starting materials, followed by a series of reactions such as epoxide opening, ring closure, and functional group transformations. Reaction conditions often involve the use of specific catalysts, solvents, and temperature control to achieve high yields and purity.
Industrial Production Methods
Industrial production of this compound may involve large-scale chemical processes with optimized reaction conditions to ensure cost-effectiveness and scalability. Techniques such as continuous flow chemistry and automated synthesis can be employed to enhance efficiency and reproducibility.
Chemical Reactions Analysis
Types of Reactions
(2R,4S)-4,5-diamino-2-fluoro-5-oxopentanoic acid can undergo various types of chemical reactions, including:
Oxidation: The compound can be oxidized to form corresponding oxo derivatives.
Reduction: Reduction reactions can convert the oxo group to hydroxyl or amino groups.
Substitution: The amino and fluoro groups can participate in nucleophilic substitution reactions.
Common Reagents and Conditions
Common reagents used in these reactions include oxidizing agents (e.g., potassium permanganate), reducing agents (e.g., sodium borohydride), and nucleophiles (e.g., amines, thiols). Reaction conditions such as pH, temperature, and solvent choice play a crucial role in determining the reaction outcome.
Major Products Formed
The major products formed from these reactions depend on the specific reagents and conditions used. For example, oxidation may yield oxo derivatives, while reduction can produce hydroxyl or amino derivatives. Substitution reactions can lead to the formation of various substituted analogs.
Scientific Research Applications
Chemistry
In chemistry, (2R,4S)-4,5-diamino-2-fluoro-5-oxopentanoic acid can be used as a building block for the synthesis of more complex molecules. Its unique functional groups make it a valuable intermediate in organic synthesis.
Biology
In biological research, this compound can be used to study enzyme-substrate interactions, protein-ligand binding, and metabolic pathways. Its structural features allow it to mimic natural substrates and inhibitors.
Medicine
In medicine, this compound has potential applications as a drug candidate or a pharmacological tool. Its ability to interact with biological targets makes it a promising compound for drug discovery and development.
Industry
In the industrial sector, this compound can be used in the production of specialty chemicals, agrochemicals, and materials science. Its reactivity and versatility make it suitable for various industrial applications.
Mechanism of Action
The mechanism of action of (2R,4S)-4,5-diamino-2-fluoro-5-oxopentanoic acid involves its interaction with specific molecular targets, such as enzymes, receptors, or nucleic acids. The compound’s functional groups enable it to form covalent or non-covalent bonds with these targets, modulating their activity and function. The pathways involved may include inhibition of enzyme activity, alteration of signal transduction, or interference with metabolic processes.
Comparison with Similar Compounds
Similar Compounds
- (2R,4S)-4-hydroxy-2-fluoro-5-oxopentanoic acid
- (2R,4S)-4,5-diamino-2-chloro-5-oxopentanoic acid
- (2R,4S)-4,5-diamino-2-bromo-5-oxopentanoic acid
Uniqueness
Compared to similar compounds, (2R,4S)-4,5-diamino-2-fluoro-5-oxopentanoic acid is unique due to the presence of both amino and fluoro groups. This combination of functional groups imparts distinct chemical properties and reactivity, making it a valuable compound for various applications. The fluoro group, in particular, enhances the compound’s stability and bioavailability, which can be advantageous in medicinal chemistry.
Properties
Molecular Formula |
C5H9FN2O3 |
---|---|
Molecular Weight |
164.14 g/mol |
IUPAC Name |
(2R,4S)-4,5-diamino-2-fluoro-5-oxopentanoic acid |
InChI |
InChI=1S/C5H9FN2O3/c6-2(5(10)11)1-3(7)4(8)9/h2-3H,1,7H2,(H2,8,9)(H,10,11)/t2-,3+/m1/s1 |
InChI Key |
VNARKFMVXYXNIO-GBXIJSLDSA-N |
Isomeric SMILES |
C([C@@H](C(=O)N)N)[C@H](C(=O)O)F |
Canonical SMILES |
C(C(C(=O)N)N)C(C(=O)O)F |
Origin of Product |
United States |
Disclaimer and Information on In-Vitro Research Products
Please be aware that all articles and product information presented on BenchChem are intended solely for informational purposes. The products available for purchase on BenchChem are specifically designed for in-vitro studies, which are conducted outside of living organisms. In-vitro studies, derived from the Latin term "in glass," involve experiments performed in controlled laboratory settings using cells or tissues. It is important to note that these products are not categorized as medicines or drugs, and they have not received approval from the FDA for the prevention, treatment, or cure of any medical condition, ailment, or disease. We must emphasize that any form of bodily introduction of these products into humans or animals is strictly prohibited by law. It is essential to adhere to these guidelines to ensure compliance with legal and ethical standards in research and experimentation.