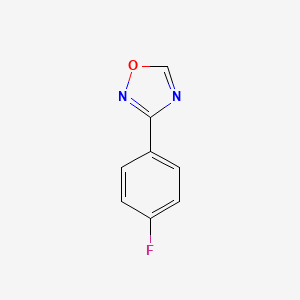
3-(4-Fluorophenyl)-1,2,4-oxadiazole
Overview
Description
3-(4-Fluorophenyl)-1,2,4-oxadiazole is a heterocyclic compound that contains a 1,2,4-oxadiazole ring substituted with a 4-fluorophenyl group. This compound is of significant interest in medicinal chemistry due to its potential biological activities and its role as a building block in the synthesis of various pharmaceuticals.
Preparation Methods
Synthetic Routes and Reaction Conditions
The synthesis of 3-(4-Fluorophenyl)-1,2,4-oxadiazole typically involves the cyclization of appropriate precursors. One common method is the reaction of 4-fluorobenzohydrazide with cyanogen bromide in the presence of a base, such as sodium hydroxide, to form the oxadiazole ring . The reaction is usually carried out under reflux conditions to ensure complete cyclization.
Industrial Production Methods
Industrial production of this compound may involve similar synthetic routes but on a larger scale. The use of continuous flow reactors and optimized reaction conditions can enhance yield and purity. Additionally, the use of microwave irradiation has been explored to reduce reaction times and improve efficiency .
Chemical Reactions Analysis
Types of Reactions
3-(4-Fluorophenyl)-1,2,4-oxadiazole can undergo various chemical reactions, including:
Substitution Reactions: The fluorine atom on the phenyl ring can be substituted with other nucleophiles under appropriate conditions.
Oxidation and Reduction: The oxadiazole ring can participate in redox reactions, although these are less common.
Common Reagents and Conditions
Substitution: Reagents such as sodium methoxide or potassium tert-butoxide can be used for nucleophilic aromatic substitution.
Oxidation: Strong oxidizing agents like potassium permanganate can be used, although care must be taken to avoid ring cleavage.
Major Products
Substitution: Products typically include derivatives where the fluorine atom is replaced by other functional groups.
Oxidation: Oxidation products may include ring-opened compounds or further oxidized derivatives.
Scientific Research Applications
Mechanism of Action
The biological activity of 3-(4-Fluorophenyl)-1,2,4-oxadiazole is attributed to its ability to interact with various molecular targets. The compound can bind to enzymes and receptors, modulating their activity. For example, it may inhibit specific enzymes involved in inflammatory pathways, thereby exerting anti-inflammatory effects . The exact molecular targets and pathways can vary depending on the specific biological context.
Comparison with Similar Compounds
Similar Compounds
- 3-(4-Chlorophenyl)-1,2,4-oxadiazole
- 3-(4-Bromophenyl)-1,2,4-oxadiazole
- 3-(4-Methylphenyl)-1,2,4-oxadiazole
Uniqueness
3-(4-Fluorophenyl)-1,2,4-oxadiazole is unique due to the presence of the fluorine atom, which imparts distinct electronic properties and influences its reactivity and biological activity. Fluorine’s high electronegativity and small size can enhance the compound’s ability to interact with biological targets, making it a valuable scaffold in drug design .
Properties
IUPAC Name |
3-(4-fluorophenyl)-1,2,4-oxadiazole | |
---|---|---|
Source | PubChem | |
URL | https://pubchem.ncbi.nlm.nih.gov | |
Description | Data deposited in or computed by PubChem | |
InChI |
InChI=1S/C8H5FN2O/c9-7-3-1-6(2-4-7)8-10-5-12-11-8/h1-5H | |
Source | PubChem | |
URL | https://pubchem.ncbi.nlm.nih.gov | |
Description | Data deposited in or computed by PubChem | |
InChI Key |
NHIDNLOFDCZWKS-UHFFFAOYSA-N | |
Source | PubChem | |
URL | https://pubchem.ncbi.nlm.nih.gov | |
Description | Data deposited in or computed by PubChem | |
Canonical SMILES |
C1=CC(=CC=C1C2=NOC=N2)F | |
Source | PubChem | |
URL | https://pubchem.ncbi.nlm.nih.gov | |
Description | Data deposited in or computed by PubChem | |
Molecular Formula |
C8H5FN2O | |
Source | PubChem | |
URL | https://pubchem.ncbi.nlm.nih.gov | |
Description | Data deposited in or computed by PubChem | |
DSSTOX Substance ID |
DTXSID80597685 | |
Record name | 3-(4-Fluorophenyl)-1,2,4-oxadiazole | |
Source | EPA DSSTox | |
URL | https://comptox.epa.gov/dashboard/DTXSID80597685 | |
Description | DSSTox provides a high quality public chemistry resource for supporting improved predictive toxicology. | |
Molecular Weight |
164.14 g/mol | |
Source | PubChem | |
URL | https://pubchem.ncbi.nlm.nih.gov | |
Description | Data deposited in or computed by PubChem | |
CAS No. |
887763-94-2 | |
Record name | 3-(4-Fluorophenyl)-1,2,4-oxadiazole | |
Source | EPA DSSTox | |
URL | https://comptox.epa.gov/dashboard/DTXSID80597685 | |
Description | DSSTox provides a high quality public chemistry resource for supporting improved predictive toxicology. | |
Retrosynthesis Analysis
AI-Powered Synthesis Planning: Our tool employs the Template_relevance Pistachio, Template_relevance Bkms_metabolic, Template_relevance Pistachio_ringbreaker, Template_relevance Reaxys, Template_relevance Reaxys_biocatalysis model, leveraging a vast database of chemical reactions to predict feasible synthetic routes.
One-Step Synthesis Focus: Specifically designed for one-step synthesis, it provides concise and direct routes for your target compounds, streamlining the synthesis process.
Accurate Predictions: Utilizing the extensive PISTACHIO, BKMS_METABOLIC, PISTACHIO_RINGBREAKER, REAXYS, REAXYS_BIOCATALYSIS database, our tool offers high-accuracy predictions, reflecting the latest in chemical research and data.
Strategy Settings
Precursor scoring | Relevance Heuristic |
---|---|
Min. plausibility | 0.01 |
Model | Template_relevance |
Template Set | Pistachio/Bkms_metabolic/Pistachio_ringbreaker/Reaxys/Reaxys_biocatalysis |
Top-N result to add to graph | 6 |
Feasible Synthetic Routes
Disclaimer and Information on In-Vitro Research Products
Please be aware that all articles and product information presented on BenchChem are intended solely for informational purposes. The products available for purchase on BenchChem are specifically designed for in-vitro studies, which are conducted outside of living organisms. In-vitro studies, derived from the Latin term "in glass," involve experiments performed in controlled laboratory settings using cells or tissues. It is important to note that these products are not categorized as medicines or drugs, and they have not received approval from the FDA for the prevention, treatment, or cure of any medical condition, ailment, or disease. We must emphasize that any form of bodily introduction of these products into humans or animals is strictly prohibited by law. It is essential to adhere to these guidelines to ensure compliance with legal and ethical standards in research and experimentation.