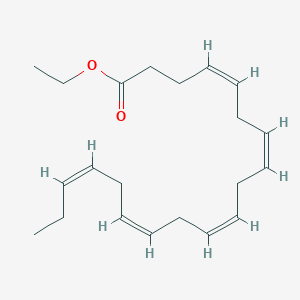
Ethyl (4Z,7Z,10Z,13Z,16Z)-Nonadeca-4,7,10,13,16--pentaenoate
- Click on QUICK INQUIRY to receive a quote from our team of experts.
- With the quality product at a COMPETITIVE price, you can focus more on your research.
Overview
Description
Ethyl (4Z,7Z,10Z,13Z,16Z)-Nonadeca-4,7,10,13,16-pentaenoate is a long-chain fatty acid ethyl ester. This compound is characterized by its multiple conjugated double bonds, which contribute to its unique chemical properties. It is a derivative of nonadeca-pentaenoic acid, where the carboxy group of the acid is esterified with ethanol.
Preparation Methods
Synthetic Routes and Reaction Conditions
The synthesis of Ethyl (4Z,7Z,10Z,13Z,16Z)-Nonadeca-4,7,10,13,16-pentaenoate typically involves the esterification of nonadeca-pentaenoic acid with ethanol. This reaction is catalyzed by an acid catalyst such as sulfuric acid or p-toluenesulfonic acid. The reaction is carried out under reflux conditions to ensure complete esterification. The product is then purified by distillation or recrystallization.
Industrial Production Methods
In an industrial setting, the production of Ethyl (4Z,7Z,10Z,13Z,16Z)-Nonadeca-4,7,10,13,16-pentaenoate involves large-scale esterification processes. The reaction is conducted in large reactors with continuous stirring and heating. The use of a continuous distillation setup helps in the removal of water formed during the reaction, driving the equilibrium towards the formation of the ester. The final product is obtained through distillation and purification processes.
Chemical Reactions Analysis
Types of Reactions
Ethyl (4Z,7Z,10Z,13Z,16Z)-Nonadeca-4,7,10,13,16-pentaenoate undergoes various chemical reactions, including:
Oxidation: The compound can be oxidized to form corresponding epoxides or hydroxylated derivatives.
Reduction: Reduction reactions can convert the double bonds to single bonds, forming saturated esters.
Substitution: The ester group can undergo nucleophilic substitution reactions, leading to the formation of different derivatives.
Common Reagents and Conditions
Oxidation: Common oxidizing agents include potassium permanganate (KMnO4) and osmium tetroxide (OsO4).
Reduction: Hydrogenation using catalysts like palladium on carbon (Pd/C) is commonly employed.
Substitution: Nucleophiles such as amines or alcohols can be used under basic or acidic conditions.
Major Products
Oxidation: Epoxides, diols, and hydroxylated esters.
Reduction: Saturated ethyl nonadecanoate.
Substitution: Amino or alkoxy derivatives of the ester.
Scientific Research Applications
Ethyl (4Z,7Z,10Z,13Z,16Z)-Nonadeca-4,7,10,13,16-pentaenoate has several applications in scientific research:
Chemistry: Used as a precursor in the synthesis of complex organic molecules and polymers.
Biology: Studied for its role in cell membrane structure and function due to its fatty acid nature.
Medicine: Investigated for its potential anti-inflammatory and antioxidant properties.
Industry: Utilized in the production of bio-based lubricants and surfactants.
Mechanism of Action
The mechanism of action of Ethyl (4Z,7Z,10Z,13Z,16Z)-Nonadeca-4,7,10,13,16-pentaenoate involves its interaction with cellular membranes and enzymes. The compound can integrate into lipid bilayers, affecting membrane fluidity and function. It may also interact with enzymes involved in lipid metabolism, modulating their activity and influencing cellular processes.
Comparison with Similar Compounds
Ethyl (4Z,7Z,10Z,13Z,16Z)-Nonadeca-4,7,10,13,16-pentaenoate can be compared with other long-chain fatty acid ethyl esters such as:
Ethyl (4Z,7Z,10Z,13Z,16Z,19Z)-docosahexaenoate: Similar in structure but with an additional double bond, making it more unsaturated.
Ethyl (4Z,7Z,10Z,13Z,16Z)-docosapentaenoate: Similar in structure but with a longer carbon chain, affecting its physical properties.
The uniqueness of Ethyl (4Z,7Z,10Z,13Z,16Z)-Nonadeca-4,7,10,13,16-pentaenoate lies in its specific chain length and degree of unsaturation, which influence its chemical reactivity and biological functions.
Properties
CAS No. |
2692622-82-3 |
---|---|
Molecular Formula |
C21H32O2 |
Molecular Weight |
316.5 g/mol |
IUPAC Name |
ethyl (4Z,7Z,10Z,13Z,16Z)-nonadeca-4,7,10,13,16-pentaenoate |
InChI |
InChI=1S/C21H32O2/c1-3-5-6-7-8-9-10-11-12-13-14-15-16-17-18-19-20-21(22)23-4-2/h5-6,8-9,11-12,14-15,17-18H,3-4,7,10,13,16,19-20H2,1-2H3/b6-5-,9-8-,12-11-,15-14-,18-17- |
InChI Key |
OITJCKVSVNJHQO-AAQCHOMXSA-N |
Isomeric SMILES |
CC/C=C\C/C=C\C/C=C\C/C=C\C/C=C\CCC(=O)OCC |
Canonical SMILES |
CCC=CCC=CCC=CCC=CCC=CCCC(=O)OCC |
Origin of Product |
United States |
Disclaimer and Information on In-Vitro Research Products
Please be aware that all articles and product information presented on BenchChem are intended solely for informational purposes. The products available for purchase on BenchChem are specifically designed for in-vitro studies, which are conducted outside of living organisms. In-vitro studies, derived from the Latin term "in glass," involve experiments performed in controlled laboratory settings using cells or tissues. It is important to note that these products are not categorized as medicines or drugs, and they have not received approval from the FDA for the prevention, treatment, or cure of any medical condition, ailment, or disease. We must emphasize that any form of bodily introduction of these products into humans or animals is strictly prohibited by law. It is essential to adhere to these guidelines to ensure compliance with legal and ethical standards in research and experimentation.