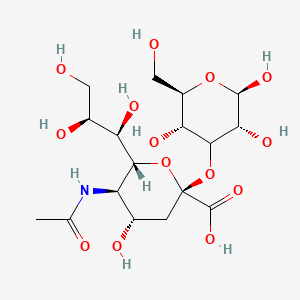
3-Sialyl-D-glucose (alpha/beta mixture)
- Click on QUICK INQUIRY to receive a quote from our team of experts.
- With the quality product at a COMPETITIVE price, you can focus more on your research.
Overview
Description
3-Sialyl-D-glucose (alpha/beta mixture) is a biochemical compound with the molecular formula C17H29NO14 and a molecular weight of 471.41. It is primarily used in proteomics research and is known for its role in various biochemical processes .
Preparation Methods
Synthetic Routes and Reaction Conditions
The synthesis of 3-Sialyl-D-glucose (alpha/beta mixture) involves the glycosylation of sialic acid with glucose. This process typically requires the use of a glycosyl donor and acceptor, along with a catalyst to facilitate the reaction. The reaction conditions often include:
Temperature: The reaction is usually carried out at low temperatures to prevent decomposition of the reactants.
Solvent: Common solvents used include dichloromethane or acetonitrile.
Catalyst: Lewis acids such as boron trifluoride etherate are often used as catalysts.
Industrial Production Methods
Industrial production of 3-Sialyl-D-glucose (alpha/beta mixture) follows similar synthetic routes but on a larger scale. The process is optimized for higher yields and purity, often involving:
Batch reactors: For controlled reaction conditions.
Purification steps: Including crystallization and chromatography to ensure high purity of the final product.
Chemical Reactions Analysis
Types of Reactions
3-Sialyl-D-glucose (alpha/beta mixture) undergoes various chemical reactions, including:
Oxidation: It can be oxidized to form corresponding acids.
Reduction: Reduction reactions can convert it into alcohol derivatives.
Substitution: It can undergo substitution reactions where functional groups are replaced with others.
Common Reagents and Conditions
Oxidizing agents: Such as potassium permanganate or chromium trioxide.
Reducing agents: Like sodium borohydride or lithium aluminum hydride.
Solvents: Including water, methanol, or ethanol.
Major Products
The major products formed from these reactions include:
Oxidized derivatives: Such as sialic acid derivatives.
Reduced derivatives: Including various alcohol forms.
Substituted derivatives: Depending on the substituent introduced.
Scientific Research Applications
3-Sialyl-D-glucose (alpha/beta mixture) has a wide range of applications in scientific research:
Chemistry: Used as a building block in the synthesis of more complex molecules.
Biology: Plays a role in studying glycosylation processes and protein interactions.
Medicine: Investigated for its potential in drug development and therapeutic applications.
Industry: Utilized in the production of biochemical reagents and diagnostic tools.
Mechanism of Action
The mechanism of action of 3-Sialyl-D-glucose (alpha/beta mixture) involves its interaction with specific molecular targets and pathways:
Molecular Targets: It interacts with glycoproteins and glycolipids, influencing their structure and function.
Pathways: It is involved in glycosylation pathways, affecting protein folding, stability, and cell signaling.
Comparison with Similar Compounds
Similar Compounds
2-Sialyl-D-glucose: Another sialylated glucose derivative with different glycosidic linkage.
3-Sialyl-D-galactose: Similar structure but with galactose instead of glucose.
3-Sialyl-D-mannose: Mannose-based sialylated compound.
Uniqueness
3-Sialyl-D-glucose (alpha/beta mixture) is unique due to its specific glycosidic linkage and the presence of both alpha and beta anomers. This duality provides it with distinct biochemical properties and applications compared to its analogs .
Biological Activity
3-Sialyl-D-glucose (α/β mixture) is a sialic acid derivative that plays a significant role in various biological processes, particularly in cell signaling, immune response, and cancer progression. This article explores its biological activity through a detailed examination of its mechanisms, implications in health and disease, and relevant research findings.
Overview of 3-Sialyl-D-glucose
3-Sialyl-D-glucose is characterized by the presence of sialic acid linked to glucose, which can exist in both α and β anomeric forms. Its molecular formula is C17H29NO14 with a molecular weight of approximately 471.41 g/mol . The compound is primarily studied for its role in glycosylation processes and its interactions with various biological molecules.
1. Sialylation and Cell Signaling:
Sialylation, the addition of sialic acid to glycoproteins or glycolipids, is crucial for modulating cell-cell interactions and signaling pathways. Research indicates that 3-sialyl-D-glucose can influence the activity of specific sialyltransferases, which are enzymes that catalyze the transfer of sialic acid to acceptor substrates. For instance, α2,3-sialyltransferases have been shown to modify integrins and other proteins involved in cell adhesion and migration .
2. Role in Cancer Progression:
Sialylation has been linked to tumor metastasis and immune evasion. Studies demonstrate that increased levels of sialylated glycans, including those containing 3-sialyl-D-glucose, correlate with enhanced metastatic potential in various cancers such as gastric and ovarian cancer . Specifically, hypersialylation promotes tumor cell survival and invasion by modifying the interaction between cancer cells and the immune system.
Case Studies
1. Sialylation in Gastric Cancer:
A study by Gomes et al. found that overexpression of ST3Gal IV, an enzyme responsible for α-2,3-sialylation, led to increased synthesis of sLe x antigen in gastric cancer cells (MKN45), enhancing their invasive phenotype both in vitro and in vivo . This suggests that targeting sialylation pathways may provide therapeutic avenues for managing gastric cancer.
2. Sialylation and Epithelial-Mesenchymal Transition (EMT):
Research conducted by Wu et al. revealed that TGF-β treatment increases ST3Gal I expression in ovarian cancer cells, leading to alterations in cellular adhesion markers such as E-cadherin and N-cadherin. This shift indicates a role for 3-sialyl-D-glucose in promoting EMT, a critical process in cancer metastasis .
Data Tables
Study | Findings | Implications |
---|---|---|
Gomes et al. (2020) | Increased ST3Gal IV leads to higher sLe x synthesis | Correlates with enhanced tumor invasiveness |
Wu et al. (2020) | TGF-β increases ST3Gal I expression affecting adhesion markers | Suggests involvement in EMT during cancer progression |
Qi et al. (2020) | Identified distinct α-2,3-sialyltransferases modifying integrins | Highlights specificity of sialylation in regulating cellular functions |
Properties
Molecular Formula |
C17H29NO14 |
---|---|
Molecular Weight |
471.4 g/mol |
IUPAC Name |
(2R,4S,5R,6R)-5-acetamido-4-hydroxy-2-[(2R,3R,5R,6R)-2,3,5-trihydroxy-6-(hydroxymethyl)oxan-4-yl]oxy-6-[(1R,2S)-1,2,3-trihydroxypropyl]oxane-2-carboxylic acid |
InChI |
InChI=1S/C17H29NO14/c1-5(21)18-9-6(22)2-17(16(28)29,31-13(9)10(24)7(23)3-19)32-14-11(25)8(4-20)30-15(27)12(14)26/h6-15,19-20,22-27H,2-4H2,1H3,(H,18,21)(H,28,29)/t6-,7-,8+,9+,10+,11+,12+,13+,14?,15+,17+/m0/s1 |
InChI Key |
GKHDMBQTTHCDCR-FCLOCSCXSA-N |
Isomeric SMILES |
CC(=O)N[C@@H]1[C@H](C[C@](O[C@H]1[C@@H]([C@H](CO)O)O)(C(=O)O)OC2[C@@H]([C@H](O[C@H]([C@@H]2O)O)CO)O)O |
Canonical SMILES |
CC(=O)NC1C(CC(OC1C(C(CO)O)O)(C(=O)O)OC2C(C(OC(C2O)O)CO)O)O |
Origin of Product |
United States |
Disclaimer and Information on In-Vitro Research Products
Please be aware that all articles and product information presented on BenchChem are intended solely for informational purposes. The products available for purchase on BenchChem are specifically designed for in-vitro studies, which are conducted outside of living organisms. In-vitro studies, derived from the Latin term "in glass," involve experiments performed in controlled laboratory settings using cells or tissues. It is important to note that these products are not categorized as medicines or drugs, and they have not received approval from the FDA for the prevention, treatment, or cure of any medical condition, ailment, or disease. We must emphasize that any form of bodily introduction of these products into humans or animals is strictly prohibited by law. It is essential to adhere to these guidelines to ensure compliance with legal and ethical standards in research and experimentation.