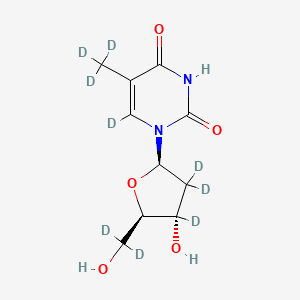
Thymidine-d9
- Click on QUICK INQUIRY to receive a quote from our team of experts.
- With the quality product at a COMPETITIVE price, you can focus more on your research.
Overview
Description
Thymidine-d9 is a deuterated form of thymidine, a nucleoside that plays a crucial role in the structure and function of DNA. Thymidine consists of the pyrimidine base thymine attached to a deoxyribose sugar. The “d9” designation indicates that nine hydrogen atoms in the molecule have been replaced with deuterium, a stable isotope of hydrogen. This modification is often used in scientific research to study metabolic pathways and molecular interactions due to the unique properties of deuterium.
Preparation Methods
Synthetic Routes and Reaction Conditions: The synthesis of Thymidine-d9 typically involves the incorporation of deuterium into the thymidine molecule. One common method is the catalytic exchange of hydrogen atoms with deuterium in the presence of a deuterium source, such as deuterium oxide (D2O). This process can be facilitated by using a palladium catalyst under specific temperature and pressure conditions.
Industrial Production Methods: Industrial production of this compound follows similar principles but on a larger scale. The process involves the use of high-purity deuterium sources and advanced catalytic systems to ensure efficient and consistent incorporation of deuterium. The final product is then purified using techniques such as chromatography to achieve the desired level of deuteration.
Chemical Reactions Analysis
Types of Reactions: Thymidine-d9 undergoes various chemical reactions similar to its non-deuterated counterpart. These include:
Oxidation: this compound can be oxidized to form thymine and other oxidation products.
Reduction: Reduction reactions can convert this compound into its corresponding deoxyribose derivatives.
Substitution: this compound can participate in nucleophilic substitution reactions, where the deuterium atoms may be replaced by other functional groups.
Common Reagents and Conditions:
Oxidation: Common oxidizing agents include potassium permanganate (KMnO4) and hydrogen peroxide (H2O2).
Reduction: Reducing agents such as sodium borohydride (NaBH4) and lithium aluminum hydride (LiAlH4) are often used.
Substitution: Nucleophilic reagents like sodium azide (NaN3) and thiols can be employed under mild conditions.
Major Products: The major products formed from these reactions depend on the specific reagents and conditions used. For example, oxidation of this compound can yield thymine, while reduction can produce deoxyribose derivatives.
Scientific Research Applications
Thymidine-d9 has a wide range of applications in scientific research, including:
Chemistry: Used as a tracer in metabolic studies to investigate the pathways and mechanisms of nucleoside metabolism.
Biology: Employed in cell proliferation assays to measure DNA synthesis and cell division rates.
Medicine: Utilized in pharmacokinetic studies to understand the absorption, distribution, metabolism, and excretion of nucleoside analogs.
Industry: Applied in the development of deuterated drugs, which often exhibit improved pharmacokinetic properties compared to their non-deuterated counterparts.
Mechanism of Action
The mechanism of action of Thymidine-d9 is similar to that of thymidine. It is incorporated into DNA during the S phase of the cell cycle, where it pairs with deoxyadenosine (A) in the DNA double helix. The presence of deuterium atoms can influence the stability and interactions of the DNA molecule, making this compound a valuable tool for studying DNA dynamics and interactions.
Molecular Targets and Pathways: this compound targets the DNA synthesis pathway and is involved in the replication and repair processes. It is phosphorylated by thymidine kinase to form thymidine monophosphate (TMP), which is further phosphorylated to thymidine diphosphate (TDP) and thymidine triphosphate (TTP). These phosphorylated forms are then incorporated into the DNA strand by DNA polymerase.
Comparison with Similar Compounds
Thymidine-d9 can be compared to other deuterated nucleosides and non-deuterated thymidine analogs:
Deuterated Nucleosides: Compounds like deuterated cytidine (Cytidine-d5) and deuterated adenosine (Adenosine-d5) are similar in that they also contain deuterium atoms. this compound is unique in its specific application to thymidine metabolism and DNA synthesis studies.
Non-Deuterated Thymidine Analogs: Thymidine analogs such as azidothymidine (AZT) and bromodeoxyuridine (BrdU) are used in antiviral and cancer research. This compound differs in that it is primarily used as a research tool to study metabolic pathways and molecular interactions.
Properties
Molecular Formula |
C10H14N2O5 |
---|---|
Molecular Weight |
251.28 g/mol |
IUPAC Name |
6-deuterio-1-[(2R,4S,5R)-3,3,4-trideuterio-5-[dideuterio(hydroxy)methyl]-4-hydroxyoxolan-2-yl]-5-(trideuteriomethyl)pyrimidine-2,4-dione |
InChI |
InChI=1S/C10H14N2O5/c1-5-3-12(10(16)11-9(5)15)8-2-6(14)7(4-13)17-8/h3,6-8,13-14H,2,4H2,1H3,(H,11,15,16)/t6-,7+,8+/m0/s1/i1D3,2D2,3D,4D2,6D |
InChI Key |
IQFYYKKMVGJFEH-LREJENERSA-N |
Isomeric SMILES |
[2H]C1=C(C(=O)NC(=O)N1[C@H]2C([C@]([C@H](O2)C([2H])([2H])O)([2H])O)([2H])[2H])C([2H])([2H])[2H] |
Canonical SMILES |
CC1=CN(C(=O)NC1=O)C2CC(C(O2)CO)O |
Origin of Product |
United States |
Disclaimer and Information on In-Vitro Research Products
Please be aware that all articles and product information presented on BenchChem are intended solely for informational purposes. The products available for purchase on BenchChem are specifically designed for in-vitro studies, which are conducted outside of living organisms. In-vitro studies, derived from the Latin term "in glass," involve experiments performed in controlled laboratory settings using cells or tissues. It is important to note that these products are not categorized as medicines or drugs, and they have not received approval from the FDA for the prevention, treatment, or cure of any medical condition, ailment, or disease. We must emphasize that any form of bodily introduction of these products into humans or animals is strictly prohibited by law. It is essential to adhere to these guidelines to ensure compliance with legal and ethical standards in research and experimentation.