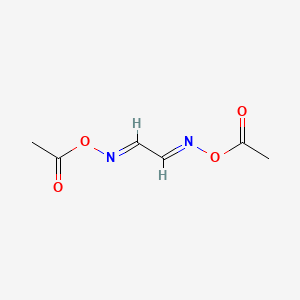
N,N'-Diacetylglyoxime
- Click on QUICK INQUIRY to receive a quote from our team of experts.
- With the quality product at a COMPETITIVE price, you can focus more on your research.
Overview
Description
N,N’-Diacetylglyoxime is an organic compound with the molecular formula C6H8N2O4. It is a derivative of glyoxime, where both oxime groups are acetylated. This compound is known for its role in coordination chemistry and its applications in various scientific fields.
Preparation Methods
Synthetic Routes and Reaction Conditions
N,N’-Diacetylglyoxime can be synthesized through the acetylation of glyoxime. The process typically involves the reaction of glyoxime with acetic anhydride in the presence of a catalyst such as pyridine. The reaction is carried out under reflux conditions to ensure complete acetylation.
Industrial Production Methods
While specific industrial production methods for N,N’-Diacetylglyoxime are not widely documented, the general approach involves large-scale acetylation reactions using optimized conditions to maximize yield and purity. The use of continuous flow reactors and advanced purification techniques can enhance the efficiency of the production process.
Chemical Reactions Analysis
Types of Reactions
N,N’-Diacetylglyoxime undergoes various chemical reactions, including:
Oxidation: It can be oxidized to form corresponding oxime derivatives.
Reduction: Reduction reactions can convert it back to glyoxime.
Substitution: The acetyl groups can be substituted with other functional groups under appropriate conditions.
Common Reagents and Conditions
Oxidation: Common oxidizing agents include hydrogen peroxide and potassium permanganate.
Reduction: Reducing agents such as sodium borohydride or lithium aluminum hydride are used.
Substitution: Reagents like alkyl halides or acyl chlorides can be used for substitution reactions.
Major Products
Oxidation: Oxime derivatives.
Reduction: Glyoxime.
Substitution: Various substituted glyoxime derivatives depending on the reagents used.
Scientific Research Applications
N,N’-Diacetylglyoxime has several applications in scientific research:
Chemistry: It is used as a ligand in coordination chemistry to form complexes with metals.
Biology: It is studied for its potential biological activities and interactions with biomolecules.
Medicine: Research is ongoing to explore its potential therapeutic applications.
Industry: It is used in the synthesis of other chemical compounds and materials.
Mechanism of Action
The mechanism of action of N,N’-Diacetylglyoxime involves its ability to form stable complexes with metal ions. The acetylated oxime groups act as chelating agents, binding to metal ions and forming coordination complexes. These complexes can exhibit various chemical and biological activities depending on the metal ion and the specific structure of the complex.
Comparison with Similar Compounds
Similar Compounds
Dimethylglyoxime: A similar compound with methyl groups instead of acetyl groups.
Glyoxime: The parent compound without acetylation.
Diacetylmonoxime: A related compound with one oxime group acetylated.
Uniqueness
N,N’-Diacetylglyoxime is unique due to its acetylated oxime groups, which enhance its stability and reactivity in forming metal complexes. This makes it particularly useful in coordination chemistry and various applications where stable metal complexes are required.
Properties
Molecular Formula |
C6H8N2O4 |
---|---|
Molecular Weight |
172.14 g/mol |
IUPAC Name |
[(E)-[(2E)-2-acetyloxyiminoethylidene]amino] acetate |
InChI |
InChI=1S/C6H8N2O4/c1-5(9)11-7-3-4-8-12-6(2)10/h3-4H,1-2H3/b7-3+,8-4+ |
InChI Key |
KEHBFQDJKKVCJK-FCXRPNKRSA-N |
Isomeric SMILES |
CC(=O)O/N=C/C=N/OC(=O)C |
Canonical SMILES |
CC(=O)ON=CC=NOC(=O)C |
Origin of Product |
United States |
Disclaimer and Information on In-Vitro Research Products
Please be aware that all articles and product information presented on BenchChem are intended solely for informational purposes. The products available for purchase on BenchChem are specifically designed for in-vitro studies, which are conducted outside of living organisms. In-vitro studies, derived from the Latin term "in glass," involve experiments performed in controlled laboratory settings using cells or tissues. It is important to note that these products are not categorized as medicines or drugs, and they have not received approval from the FDA for the prevention, treatment, or cure of any medical condition, ailment, or disease. We must emphasize that any form of bodily introduction of these products into humans or animals is strictly prohibited by law. It is essential to adhere to these guidelines to ensure compliance with legal and ethical standards in research and experimentation.