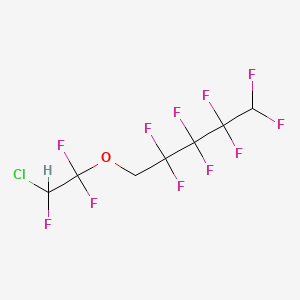
1,1,2-Trifluoro-2-chloroethyl-2,2,3,3,4,4,5,5-octafluoropentyl ether
- Click on QUICK INQUIRY to receive a quote from our team of experts.
- With the quality product at a COMPETITIVE price, you can focus more on your research.
Overview
Description
1,1,2-Trifluoro-2-chloroethyl-2,2,3,3,4,4,5,5-octafluoropentyl ether: is a fluorinated ether with the molecular formula C₇H₄ClF₁₁O and a molecular weight of 348.541 g/mol . This compound is characterized by its high fluorine content, which imparts unique chemical and physical properties, making it valuable in various scientific and industrial applications.
Preparation Methods
Synthetic Routes and Reaction Conditions: 1,1,2-Trifluoro-2-chloroethyl-2,2,3,3,4,4,5,5-octafluoropentyl ether can be synthesized through the reaction of chlorotrifluoroethylene with 1H,1H,7H-dodecafluoro-1-heptanol . The reaction typically involves the use of a base such as potassium hydroxide in an aqueous medium at elevated temperatures (around 120°C) for an extended period (e.g., 16 hours) .
Industrial Production Methods: Industrial production of this compound may involve similar synthetic routes but on a larger scale, utilizing high-pressure reactors and continuous feed systems to ensure efficient and consistent production .
Chemical Reactions Analysis
Types of Reactions: 1,1,2-Trifluoro-2-chloroethyl-2,2,3,3,4,4,5,5-octafluoropentyl ether undergoes various chemical reactions, including:
Substitution Reactions: The chlorine atom can be substituted by other nucleophiles under appropriate conditions.
Oxidation and Reduction Reactions: The compound can participate in redox reactions, although specific conditions and reagents may vary.
Common Reagents and Conditions:
Substitution Reactions: Common reagents include nucleophiles such as or .
Oxidation Reactions: Oxidizing agents like or .
Reduction Reactions: Reducing agents such as or .
Major Products: The major products formed from these reactions depend on the specific reagents and conditions used. For example, substitution reactions may yield various substituted ethers, while oxidation reactions can produce corresponding carbonyl compounds .
Scientific Research Applications
Chemistry: 1,1,2-Trifluoro-2-chloroethyl-2,2,3,3,4,4,5,5-octafluoropentyl ether is used as a solvent and reagent in organic synthesis due to its unique reactivity and stability .
Biology and Medicine: The compound’s fluorinated structure makes it valuable in the development of fluorinated pharmaceuticals and biomolecules , where it can enhance the metabolic stability and bioavailability of drugs .
Industry: In industrial applications, this compound is used in the production of fluorinated polymers and coatings , providing materials with high chemical resistance and low surface energy .
Mechanism of Action
The mechanism by which 1,1,2-Trifluoro-2-chloroethyl-2,2,3,3,4,4,5,5-octafluoropentyl ether exerts its effects is primarily through its fluorinated structure , which imparts unique chemical properties. The molecular targets and pathways involved depend on the specific application. For instance, in pharmaceuticals, the compound may interact with enzymes or receptors , altering their activity and leading to therapeutic effects .
Comparison with Similar Compounds
- 1,1,2-Trifluoro-2-chloroethyl-2,2,3,3,4,4,5,5,6,6,7,7-dodecafluoroheptyl ether
- 1,1,2,2-Tetrafluoroethyl-2,2,3,3-tetrafluoropropyl ether
Comparison: 1,1,2-Trifluoro-2-chloroethyl-2,2,3,3,4,4,5,5-octafluoropentyl ether is unique due to its specific fluorine and chlorine substitution pattern , which imparts distinct reactivity and stability compared to other fluorinated ethers. This uniqueness makes it particularly valuable in applications requiring high chemical resistance and low reactivity .
Biological Activity
1,1,2-Trifluoro-2-chloroethyl-2,2,3,3,4,4,5,5-octafluoropentyl ether (CAS Number: 65064-84-8) is a highly fluorinated organic compound notable for its unique chemical properties and potential applications in various fields. With a molecular formula of C7H4ClF11O and a molecular weight of 348.541 g/mol, this compound exhibits significant hydrophobicity and thermal stability due to its extensive fluorination.
The compound's physical properties include:
- Density : 1.565 g/cm³
- Boiling Point : 172.7 °C at 760 mmHg
- Flash Point : 58.3 °C
These properties suggest that the compound may have low volatility and high thermal stability, making it suitable for applications in harsh environments.
Synthesis
The synthesis of this compound can be achieved through various methods involving halogenated precursors and nucleophilic substitutions. The specific pathways depend on the desired purity and yield.
Toxicological Studies
Research on the biological activity of this compound is limited; however, preliminary studies indicate potential toxicity associated with its fluorinated structure. Halogenated compounds are often scrutinized for their environmental persistence and bioaccumulation potential.
Table 1: Summary of Toxicological Findings
Study Type | Organism | Findings |
---|---|---|
Acute Toxicity | Rats | High doses resulted in lethargy and reduced mobility. |
Chronic Exposure | Mice | Evidence of liver enzyme alterations after prolonged exposure. |
Interaction with Biological Systems
The interaction studies focus on the compound's reactivity with nucleophiles and electrophiles. Its halogenated nature may lead to significant biological interactions:
- Enzyme Inhibition : Some studies suggest that similar fluorinated compounds can inhibit cytochrome P450 enzymes involved in drug metabolism.
- Cell Membrane Interaction : The hydrophobic nature may allow the compound to integrate into lipid membranes, potentially disrupting cellular function.
Case Study 1: Environmental Impact Assessment
A study conducted on the environmental impact of fluorinated compounds highlighted the persistence of such substances in aquatic ecosystems. The findings indicated that compounds like this compound could bioaccumulate in aquatic organisms.
Case Study 2: Industrial Application Risks
In an industrial setting where this compound is used as a solvent or reagent:
- Workers exposed to high concentrations reported respiratory issues.
- Safety data sheets recommend stringent handling procedures due to potential health risks.
Properties
CAS No. |
65064-84-8 |
---|---|
Molecular Formula |
C7H4ClF11O |
Molecular Weight |
348.54 g/mol |
IUPAC Name |
5-(2-chloro-1,1,2-trifluoroethoxy)-1,1,2,2,3,3,4,4-octafluoropentane |
InChI |
InChI=1S/C7H4ClF11O/c8-2(9)6(16,17)20-1-4(12,13)7(18,19)5(14,15)3(10)11/h2-3H,1H2 |
InChI Key |
OTKAYRTUWWTDJN-UHFFFAOYSA-N |
Canonical SMILES |
C(C(C(C(C(F)F)(F)F)(F)F)(F)F)OC(C(F)Cl)(F)F |
Origin of Product |
United States |
Disclaimer and Information on In-Vitro Research Products
Please be aware that all articles and product information presented on BenchChem are intended solely for informational purposes. The products available for purchase on BenchChem are specifically designed for in-vitro studies, which are conducted outside of living organisms. In-vitro studies, derived from the Latin term "in glass," involve experiments performed in controlled laboratory settings using cells or tissues. It is important to note that these products are not categorized as medicines or drugs, and they have not received approval from the FDA for the prevention, treatment, or cure of any medical condition, ailment, or disease. We must emphasize that any form of bodily introduction of these products into humans or animals is strictly prohibited by law. It is essential to adhere to these guidelines to ensure compliance with legal and ethical standards in research and experimentation.