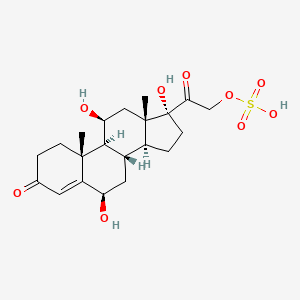
6-beta-Hydroxycortisol Sulfate
- Click on QUICK INQUIRY to receive a quote from our team of experts.
- With the quality product at a COMPETITIVE price, you can focus more on your research.
Overview
Description
6-beta-Hydroxycortisol Sulfate (CAS 53587-06-7) is a phase II sulfated metabolite of cortisol, formed through the sequential action of cytochrome P450 3A4 (CYP3A4) and sulfotransferases (SULTs). CYP3A4 hydroxylates cortisol at the 6-beta position, followed by sulfation, which enhances water solubility for renal excretion . This metabolite is used as a biomarker for assessing CYP3A4 activity in clinical and pharmacological studies, particularly in drug-drug interaction research . Its molecular weight is 458.53 g/mol, distinguishing it from non-sulfated analogs .
Preparation Methods
Synthetic Routes and Reaction Conditions: The synthesis of 6-beta-Hydroxycortisol Sulfate involves the hydroxylation of cortisol at the 6-beta position. This reaction is catalyzed by the enzyme CYP3A4 in the liver. The hydroxylated product is then sulfated to form this compound. The reaction conditions typically involve the use of specific cofactors and optimal pH levels to ensure enzyme activity .
Industrial Production Methods: Industrial production of this compound is achieved through biotechnological processes that utilize genetically engineered microorganisms expressing CYP3A4. These microorganisms are cultured in bioreactors under controlled conditions to maximize the yield of the desired product. The compound is then extracted and purified using chromatographic techniques .
Chemical Reactions Analysis
Synthetic Pathways and Precursor Reactions
6β-Hydroxycortisol sulfate is synthesized through sequential enzymatic modifications of cortisol:
-
Step 1 : Cortisol undergoes 6β-hydroxylation via CYP3A4 to form 6β-hydroxycortisol .
-
Step 2 : Sulfation of the hydroxyl group at position 21 or another position by sulfotransferases (SULTs), forming the sulfate conjugate .
Key Synthetic Parameters
Reaction Step | Enzyme Involved | Kinetic Parameter (CYP3A4) | Inhibition (IC₅₀) |
---|---|---|---|
6β-Hydroxylation of cortisol | CYP3A4 | Km=25μM | Itraconazole: 3.1nM |
Sulfation | SULT2A1 | N/A | N/A |
Degradation and Metabolic Interconversion
The sulfate conjugate undergoes reversible and irreversible transformations:
-
Hydrolysis : Removal of the sulfate group by sulfatases regenerates 6β-hydroxycortisol .
-
Oxidation-Reduction : Interconversion with 6β-hydroxycortisone via 11β-hydroxysteroid dehydrogenase (11β-HSD) .
-
Further Hydroxylation : CYP3A4-mediated oxidation at other positions (e.g., 1β or 2β) .
Metabolic Stability Data
Enzymatic Interactions and Inhibition
CYP3A4 is the primary enzyme responsible for 6β-hydroxylation, contributing >85% to cortisol metabolism . Key findings include:
-
CYP3A Inhibition : Ketoconazole reduces 6β-hydroxycortisol formation by 79–93% .
-
CYP3A Induction : Rifampicin increases urinary 6β-hydroxycortisol excretion 5-fold .
Enzyme Contribution to 6β-Hydroxylation
Enzyme | Relative Activity (vs. CYP3A4) | Substrate Specificity |
---|---|---|
CYP3A4 | 100% | Cortisol, cortisone |
CYP2B6 | <1% | Cortisol only |
CYP2D6 | <1% | Cortisone only |
Kinetic data from recombinant enzyme assays |
Analytical Detection and Quantitation
Liquid chromatography-tandem mass spectrometry (LC-MS/MS) is the gold standard for detecting 6β-hydroxycortisol sulfate:
LC-MS/MS Parameters
Analyte | Transition (m/z) | DP (V) | CE (V) |
---|---|---|---|
6β-Hydroxycortisol sulfate | 377.1 → 347.3 | -100 | -15 |
Cortisol sulfate | 361.1 → 331.2 | -40 | -15 |
Validation Metrics
Parameter | 6β-Hydroxycortisol | Cortisol |
---|---|---|
Linearity (R2) | 0.9996 | 0.9987 |
Intra-day precision (CV%) | 3.41–15.49% | 3.81–5.15% |
Inter-day precision (CV%) | 6.22–19.83% | 3.69–7.12% |
Functional Role in Drug-Drug Interactions
The urinary 6β-hydroxycortisol/cortisol ratio correlates strongly with hepatic CYP3A activity:
-
Inhibition : Ketoconazole reduces the ratio by 80%, reflecting CYP3A suppression .
-
Induction : Rifampicin increases the ratio 2–5 fold, indicating enzyme induction .
Correlation with Midazolam Clearance
CYP3A Status | 6β-Hydroxycortisol/Cortisol Ratio | Midazolam Clearance |
---|---|---|
Baseline | 1.0 | 100% |
Inhibition | 0.2 | 20% |
Induction | 2.5 | 250% |
Adapted from clinical pharmacometabolomics data |
Scientific Research Applications
6-beta-Hydroxycortisol Sulfate has several scientific research applications:
Chemistry: Used as a reference standard in analytical chemistry for the quantification of steroid metabolites.
Biology: Serves as a biomarker for studying the activity of CYP3A4 enzymes and their role in drug metabolism.
Medicine: Investigated for its potential diagnostic value in diseases related to cortisol metabolism, such as Cushing’s syndrome and Addison’s disease.
Mechanism of Action
The primary mechanism of action of 6-beta-Hydroxycortisol Sulfate involves its role as a metabolite of cortisol. The enzyme CYP3A4 catalyzes the hydroxylation of cortisol to form 6-beta-Hydroxycortisol, which is then sulfated. This process is crucial for the metabolism and clearance of cortisol from the body. The levels of this compound can be influenced by drugs that induce or inhibit CYP3A4, thereby affecting cortisol metabolism .
Comparison with Similar Compounds
Comparison with Structurally and Functionally Related Compounds
Structural Analogs of Cortisol Metabolites
The table below highlights key differences between 6-beta-Hydroxycortisol Sulfate and related cortisol derivatives:
Key Observations :
- Sulfation vs. Glucuronidation : Sulfation increases molecular weight by ~80 g/mol compared to hydroxylated cortisol, while glucuronidation adds ~156 g/mol. Sulfated metabolites are typically more polar and excreted faster than glucuronides .
- Biomarker Utility : Both 6-beta-Hydroxycortisol and its sulfate conjugate reflect CYP3A4 induction, but the sulfate form additionally depends on SULT activity, complicating its interpretation in multi-enzyme interactions .
Functional Comparison with Other Sulfated Steroids
Sulfation is a common detoxification mechanism for steroids. Notable comparisons include:
Dehydroepiandrosterone Sulfate (DHEA-S)
- Role : Major adrenal androgen precursor.
- Metabolism : Sulfated directly by SULT2A1, independent of CYP3A3.
- Clinical Use : Marker for adrenal function, contrasting with this compound’s focus on hepatic CYP3A4 .
Estrone Sulfate
- Role : Estrogen reservoir in plasma.
- Metabolism : Formed via sulfation of estrone; desulfation reactivates hormonal activity.
- Divergence : Unlike this compound, estrone sulfate serves as a circulating hormone pool rather than a metabolic endpoint .
Analytical Challenges in Detection
- This compound : Requires LC-MS/MS with negative ionization due to the sulfate group’s polarity. Cross-reactivity with glucuronides must be ruled out .
- Non-Sulfated Analogs: Detectable via positive ionization but may lack specificity in complex matrices like urine .
Research Findings and Clinical Relevance
- CYP3A4 Induction : St John’s Wort increases the urinary 6-beta-hydroxycortisol/cortisol ratio by 1.7-fold, reflecting CYP3A4 induction. The sulfate form’s levels may vary based on SULT expression .
- Drug Interactions : Bosentan (a CYP3A4 inducer) elevates 6-beta-hydroxycortisol excretion, demonstrating its utility in predicting interactions with CYP3A4 substrates like cyclosporine .
- Stability Issues : Sulfated metabolites are prone to enzymatic hydrolysis during sample preparation, necessitating rapid processing and stabilization .
Biological Activity
Overview
6-beta-Hydroxycortisol Sulfate is a significant endogenous steroid metabolite derived from cortisol, primarily formed through the enzymatic action of cytochrome P450 3A4 (CYP3A4). This compound plays a crucial role in the metabolism of corticosteroids and serves as a biomarker for assessing adrenal function and CYP3A4 activity, which is vital for drug metabolism and cortisol clearance in the human body.
Chemical Structure and Formation
The chemical formula of this compound is C21H30O6S, with a molecular weight of approximately 394.54 g/mol. Its formation involves two key processes:
- Hydroxylation : Conversion of cortisol to 6-beta-Hydroxycortisol.
- Sulfation : Addition of a sulfate group to form this compound.
These processes highlight the compound's role in corticosteroid metabolism and its utility as a metabolic marker.
The primary mechanism by which this compound operates involves its formation from cortisol via CYP3A4. This enzyme catalyzes the hydroxylation of cortisol, which is subsequently sulfated. The activity levels of this compound can be influenced by various factors, including:
- Drug Interactions : Certain medications can induce or inhibit CYP3A4, affecting cortisol metabolism and consequently altering levels of this compound.
- Physiological Conditions : Factors such as stress, fasting, and hormonal changes can also influence its levels.
Biological Significance
This compound serves multiple biological functions:
- Biomarker for CYP3A4 Activity : It is widely used to assess the functional status of CYP3A4 in clinical settings, providing insights into drug metabolism and potential drug-drug interactions.
- Indicator of Adrenal Function : Elevated levels may indicate hypercortisolism or adrenal dysfunction, while reduced levels could suggest adrenal insufficiency .
Table 1: Comparison of Urinary Excretion Rates
Condition | 6-beta-Hydroxycortisol (pmol/min) | Cortisol (pmol/min) |
---|---|---|
Healthy Controls | 40 ± 10 | 200 ± 50 |
Hypercortisolism | 120 ± 20 | 600 ± 100 |
Adrenal Insufficiency | 10 ± 5 | 50 ± 10 |
This table illustrates significant differences in urinary excretion rates between healthy individuals and those with adrenal disorders, underscoring the importance of measuring this metabolite in clinical diagnostics .
Case Study: Drug Interaction Analysis
In a study involving patients treated with rifampicin, researchers observed a marked increase in urinary excretion of 6-beta-Hydroxycortisol. The study concluded that rifampicin induced CYP3A4 activity, leading to elevated levels of this metabolite. The findings suggest that monitoring urinary levels can provide insights into drug interactions and metabolic changes induced by pharmacotherapy .
Q & A
Basic Question: What analytical methods are recommended for quantifying 6β-hydroxycortisol sulfate in biological matrices?
Methodological Answer:
Liquid chromatography-tandem mass spectrometry (LC-MS/MS) is the gold standard due to its high specificity and sensitivity for detecting sulfated metabolites in urine or plasma. Protocols should include enzymatic deconjugation (e.g., using β-glucuronidase/sulfatase) to release free metabolites prior to analysis. Internal standards like deuterated analogs (e.g., cortisol-d4) are critical for normalization . Data validation should follow FDA bioanalytical guidelines, including tests for linearity (1–100 ng/mL), precision (CV <15%), and recovery rates .
Basic Question: What is the biological significance of 6β-hydroxycortisol sulfate as a metabolic marker?
Methodological Answer:
6β-Hydroxycortisol sulfate is an endogenous biomarker of CYP3A4 enzyme activity, reflecting hepatic and intestinal metabolism of corticosteroids and xenobiotics. Its urinary excretion ratio to cortisol (6β-OHF/cortisol) is used to assess CYP3A4 induction or inhibition in drug interaction studies. Researchers should account for inter-individual variability (e.g., age, genetics) by collecting longitudinal samples and normalizing to creatinine levels .
Advanced Question: How should experimental designs control for confounding variables when assessing 6β-hydroxycortisol sulfate’s role in drug-drug interactions?
Methodological Answer:
Controlled crossover studies with washout periods are essential. For example, to evaluate CYP3A4 induction by drugs like bosentan, baseline 6β-OHF/cortisol ratios should be compared post-treatment. Confounding factors include concurrent use of CYP3A4 inhibitors (e.g., ketoconazole) or circadian rhythm effects. Randomization, stratification by genotype (e.g., CYP3A5 polymorphisms), and pharmacokinetic modeling (e.g., non-compartmental analysis) mitigate bias .
Advanced Question: How can contradictory data on 6β-hydroxycortisol sulfate stability under varying storage conditions be resolved?
Methodological Answer:
Discrepancies in stability studies often arise from differences in matrix composition (urine vs. plasma) or temperature fluctuations. A systematic approach includes:
- Testing stability at -80°C (long-term) vs. 4°C (short-term) with aliquots analyzed at 0, 24, and 72 hours.
- Adding antioxidants (e.g., ascorbic acid) to prevent sulfate ester degradation.
- Validating with spiked quality control samples and comparing degradation kinetics using Arrhenius plots .
Advanced Question: What statistical approaches are appropriate for analyzing longitudinal 6β-hydroxycortisol sulfate excretion patterns?
Methodological Answer:
Mixed-effects models are preferred to account for within-subject correlations and missing data. Key steps:
- Log-transform excretion rates to normalize skewed distributions.
- Include fixed effects (e.g., time, dose) and random effects (e.g., subject ID).
- Use likelihood ratio tests to compare nested models or AIC/BIC for model selection.
- Sensitivity analyses (e.g., bootstrapping) confirm robustness .
Basic Question: How is 6β-hydroxycortisol sulfate standardized in reference materials for clinical research?
Methodological Answer:
Certified reference materials (CRMs) from suppliers like TLC PharmaChem (ZTL-C-2718) or BTC2718 are characterized via nuclear magnetic resonance (NMR) and high-resolution MS. Researchers must verify purity (>95%) and stability through accelerated degradation studies (40°C/75% RH for 4 weeks). Batch-specific certificates of analysis should accompany all experimental data .
Advanced Question: What mechanisms explain demographic variability in 6β-hydroxycortisol sulfate excretion rates?
Methodological Answer:
Variability arises from genetic (e.g., CYP3A4*1B allele), hormonal (e.g., cortisol diurnal rhythm), and physiological factors (e.g., renal clearance efficiency). Methodological strategies:
- Stratify cohorts by age, sex, and ethnicity.
- Measure polymorphisms via PCR-RFLP or next-generation sequencing.
- Use multivariate regression to isolate covariates (e.g., glomerular filtration rate) .
Advanced Question: How can in vitro-in vivo extrapolation (IVIVE) models optimize 6β-hydroxycortisol sulfate pharmacokinetic predictions?
Methodological Answer:
IVIVE workflows integrate hepatocyte incubation data (intrinsic clearance) with physiologically based pharmacokinetic (PBPK) modeling. Key steps:
Properties
Molecular Formula |
C21H30O9S |
---|---|
Molecular Weight |
458.5 g/mol |
IUPAC Name |
[2-oxo-2-[(6R,8S,9S,10R,11S,13S,14S,17R)-6,11,17-trihydroxy-10,13-dimethyl-3-oxo-2,6,7,8,9,11,12,14,15,16-decahydro-1H-cyclopenta[a]phenanthren-17-yl]ethyl] hydrogen sulfate |
InChI |
InChI=1S/C21H30O9S/c1-19-5-3-11(22)7-14(19)15(23)8-12-13-4-6-21(26,17(25)10-30-31(27,28)29)20(13,2)9-16(24)18(12)19/h7,12-13,15-16,18,23-24,26H,3-6,8-10H2,1-2H3,(H,27,28,29)/t12-,13-,15+,16-,18+,19-,20-,21-/m0/s1 |
InChI Key |
DHXWQUUKVSNJDI-UJXAPRPESA-N |
Isomeric SMILES |
C[C@]12CCC(=O)C=C1[C@@H](C[C@@H]3[C@@H]2[C@H](C[C@]4([C@H]3CC[C@@]4(C(=O)COS(=O)(=O)O)O)C)O)O |
Canonical SMILES |
CC12CCC(=O)C=C1C(CC3C2C(CC4(C3CCC4(C(=O)COS(=O)(=O)O)O)C)O)O |
Origin of Product |
United States |
Disclaimer and Information on In-Vitro Research Products
Please be aware that all articles and product information presented on BenchChem are intended solely for informational purposes. The products available for purchase on BenchChem are specifically designed for in-vitro studies, which are conducted outside of living organisms. In-vitro studies, derived from the Latin term "in glass," involve experiments performed in controlled laboratory settings using cells or tissues. It is important to note that these products are not categorized as medicines or drugs, and they have not received approval from the FDA for the prevention, treatment, or cure of any medical condition, ailment, or disease. We must emphasize that any form of bodily introduction of these products into humans or animals is strictly prohibited by law. It is essential to adhere to these guidelines to ensure compliance with legal and ethical standards in research and experimentation.