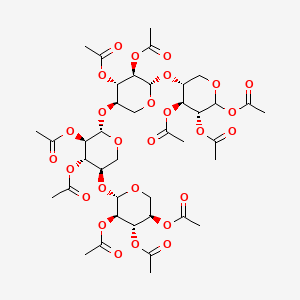
Xylotetraose Decaacetate
- Click on QUICK INQUIRY to receive a quote from our team of experts.
- With the quality product at a COMPETITIVE price, you can focus more on your research.
Overview
Description
Preparation Methods
Synthetic Routes and Reaction Conditions
Xylotetraose Decaacetate is typically synthesized through the acetylation of xylotetraose. The process involves the reaction of xylotetraose with acetic anhydride in the presence of a catalyst such as pyridine . The reaction is carried out under controlled conditions to ensure complete acetylation of all hydroxyl groups, resulting in the formation of this compound.
Industrial Production Methods
Industrial production of this compound follows similar synthetic routes but on a larger scale. The process involves the use of large reactors and precise control of reaction conditions to ensure high yield and purity of the final product . The product is then purified using techniques such as chromatography to remove any impurities.
Chemical Reactions Analysis
Types of Reactions
Xylotetraose Decaacetate undergoes various chemical reactions, including:
Hydrolysis: The compound can be hydrolyzed to yield xylotetraose and acetic acid.
Oxidation: It can undergo oxidation reactions to form corresponding oxidized derivatives.
Substitution: The acetyl groups can be substituted with other functional groups under appropriate conditions.
Common Reagents and Conditions
Hydrolysis: Typically carried out using acidic or basic conditions.
Oxidation: Common oxidizing agents include potassium permanganate and hydrogen peroxide.
Substitution: Reagents such as sodium methoxide can be used for substitution reactions.
Major Products Formed
Hydrolysis: Xylotetraose and acetic acid.
Oxidation: Oxidized derivatives of xylotetraose.
Substitution: Derivatives with substituted functional groups.
Scientific Research Applications
Xylotetraose Decaacetate has a wide range of applications in scientific research:
Chemistry: Used as an intermediate in the synthesis of other complex carbohydrates and oligosaccharides.
Biology: Employed in studies related to carbohydrate metabolism and enzyme activity.
Medicine: Investigated for its potential prebiotic effects and its role in promoting gut health.
Industry: Utilized in the production of functional foods and as a component in various industrial processes.
Mechanism of Action
The mechanism of action of Xylotetraose Decaacetate involves its interaction with specific enzymes and proteins. In biological systems, it is hydrolyzed by enzymes such as β-xylanase and β-xylosidase to release xylotetraose, which can then be further metabolized . The compound’s acetyl groups may also play a role in modulating its interactions with enzymes and other molecular targets .
Comparison with Similar Compounds
Similar Compounds
Xylobiose: A disaccharide composed of two D-xylose residues.
Xylotriose: A trisaccharide composed of three D-xylose residues.
Xylopentaose: A pentasaccharide composed of five D-xylose residues.
Uniqueness
Xylotetraose Decaacetate is unique due to its complete acetylation, which imparts distinct chemical properties and reactivity compared to its non-acetylated counterparts. This modification enhances its stability and makes it suitable for various industrial and research applications .
Biological Activity
Xylotetraose Decaacetate is a modified oligosaccharide derived from xylan, a hemicellulosic polysaccharide found in plant cell walls. This compound has garnered interest due to its potential biological activities, particularly in the context of prebiotic effects and interactions with gut microbiota. This article delves into the biological activity of this compound, supported by relevant research findings, data tables, and case studies.
Overview of this compound
Xylotetraose is a tetrasaccharide consisting of four xylose units. The decaacetate form indicates that each hydroxyl group in the xylose units has been acetylated, which may enhance its stability and bioactivity. The biological implications of such modifications are significant, particularly in terms of enzymatic hydrolysis and prebiotic potential.
Biological Activities
1. Prebiotic Effects
This compound exhibits prebiotic properties, promoting the growth of beneficial gut bacteria. Research indicates that xylooligosaccharides (XOS), which can be derived from Xylotetraose through hydrolysis, stimulate the growth of specific bacteria such as Bifidobacterium and Lactobacillus. These bacteria are known to produce short-chain fatty acids (SCFAs), which are beneficial for gut health and overall metabolism .
2. Antioxidant Activity
The antioxidant potential of this compound has been investigated through various assays. Studies have shown that oligosaccharides with higher degrees of polymerization (DP) exhibit greater antioxidant activity. However, the acetylation process may alter this activity, with unsubstituted XOS generally demonstrating superior antioxidant properties compared to their acetylated counterparts .
3. Enzymatic Hydrolysis
The enzymatic hydrolysis of this compound is crucial for its biological activity. Enzymes such as xylanases play a significant role in breaking down xylan into xylooligosaccharides. The efficiency of this process can be influenced by the presence of other polysaccharides like cellulose and lignin, which may inhibit hydrolysis .
Case Studies
- Prebiotic Potential Study
- Antioxidant Activity Assessment
Data Table: Enzymatic Hydrolysis Efficiency
Substrate | Enzyme Used | Hydrolysis Time (h) | Yield (%) |
---|---|---|---|
Xylobiose | Endo-xylanase | 24 | 70 |
Xylotriose | Exo-xylanase | 24 | 65 |
Xylotetraose | Mixed enzyme system | 24 | 75 |
This compound | Acetylated enzyme mix | 24 | 60 |
Properties
Molecular Formula |
C40H54O27 |
---|---|
Molecular Weight |
966.8 g/mol |
IUPAC Name |
[(3R,4S,5R,6S)-4,5-diacetyloxy-6-[(3R,4S,5R,6S)-4,5-diacetyloxy-6-[(3R,4S,5R,6S)-4,5-diacetyloxy-6-[(3R,4S,5R)-4,5,6-triacetyloxyoxan-3-yl]oxyoxan-3-yl]oxyoxan-3-yl]oxyoxan-3-yl] acetate |
InChI |
InChI=1S/C40H54O27/c1-15(41)55-25-11-52-38(34(61-21(7)47)29(25)56-16(2)42)66-27-13-54-40(36(63-23(9)49)31(27)58-18(4)44)67-28-14-53-39(35(62-22(8)48)32(28)59-19(5)45)65-26-12-51-37(64-24(10)50)33(60-20(6)46)30(26)57-17(3)43/h25-40H,11-14H2,1-10H3/t25-,26-,27-,28-,29+,30+,31+,32+,33-,34-,35-,36-,37?,38+,39+,40+/m1/s1 |
InChI Key |
AXGZKFVWSVOKRF-UGMHODLOSA-N |
Isomeric SMILES |
CC(=O)O[C@@H]1CO[C@H]([C@@H]([C@H]1OC(=O)C)OC(=O)C)O[C@@H]2CO[C@H]([C@@H]([C@H]2OC(=O)C)OC(=O)C)O[C@@H]3CO[C@H]([C@@H]([C@H]3OC(=O)C)OC(=O)C)O[C@@H]4COC([C@@H]([C@H]4OC(=O)C)OC(=O)C)OC(=O)C |
Canonical SMILES |
CC(=O)OC1COC(C(C1OC(=O)C)OC(=O)C)OC2COC(C(C2OC(=O)C)OC(=O)C)OC3COC(C(C3OC(=O)C)OC(=O)C)OC4COC(C(C4OC(=O)C)OC(=O)C)OC(=O)C |
Origin of Product |
United States |
Disclaimer and Information on In-Vitro Research Products
Please be aware that all articles and product information presented on BenchChem are intended solely for informational purposes. The products available for purchase on BenchChem are specifically designed for in-vitro studies, which are conducted outside of living organisms. In-vitro studies, derived from the Latin term "in glass," involve experiments performed in controlled laboratory settings using cells or tissues. It is important to note that these products are not categorized as medicines or drugs, and they have not received approval from the FDA for the prevention, treatment, or cure of any medical condition, ailment, or disease. We must emphasize that any form of bodily introduction of these products into humans or animals is strictly prohibited by law. It is essential to adhere to these guidelines to ensure compliance with legal and ethical standards in research and experimentation.