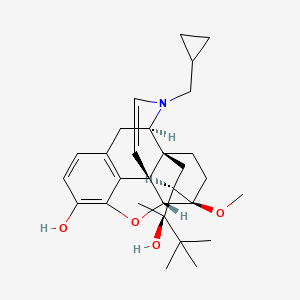
15,16-Didehydrobuprenorphine
- Click on QUICK INQUIRY to receive a quote from our team of experts.
- With the quality product at a COMPETITIVE price, you can focus more on your research.
Overview
Description
15,16-Didehydrobuprenorphine is a synthetic derivative of buprenorphine, an opioid used primarily for pain management and opioid addiction treatment. This compound is characterized by its unique chemical structure, which includes a 6,14-ethenomorphinan skeleton and a 17-(cyclopropylmethyl) group. It is known for its high affinity for opioid receptors, making it a subject of interest in pharmacological research .
Preparation Methods
The synthesis of 15,16-Didehydrobuprenorphine involves several steps, starting from the precursor buprenorphine. The key steps include:
Oxidation: Buprenorphine undergoes oxidation to form the 15,16-didehydro derivative.
Cyclization: The formation of the 6,14-ethenomorphinan skeleton is achieved through cyclization reactions.
Functional Group Modifications: Introduction of the 17-(cyclopropylmethyl) group and other functional groups is carried out under specific reaction conditions.
Industrial production methods for this compound are not widely documented, but they likely involve similar synthetic routes with optimizations for large-scale production.
Chemical Reactions Analysis
15,16-Didehydrobuprenorphine undergoes various chemical reactions, including:
Oxidation: This reaction can further modify the compound, potentially forming new derivatives.
Reduction: Reduction reactions can alter the double bonds in the structure, leading to different analogs.
Substitution: Common reagents such as halogens can be used to substitute specific functional groups in the molecule.
Hydrolysis: This reaction can break down the compound into smaller fragments under acidic or basic conditions.
The major products formed from these reactions depend on the specific reagents and conditions used.
Scientific Research Applications
15,16-Didehydrobuprenorphine has several applications in scientific research:
Pharmacology: It is used to study opioid receptor interactions and the development of new analgesics.
Medicinal Chemistry: Researchers explore its potential as a lead compound for developing new drugs with improved efficacy and safety profiles.
Neuroscience: The compound is used to investigate the mechanisms of opioid addiction and pain modulation.
Analytical Chemistry: It serves as a reference standard in various analytical techniques
Mechanism of Action
15,16-Didehydrobuprenorphine exerts its effects primarily through its interaction with opioid receptors. It acts as a partial agonist at the mu-opioid receptor and an antagonist at the kappa-opioid receptor. This dual activity results in analgesic effects while reducing the risk of respiratory depression and other side effects associated with full agonists .
Comparison with Similar Compounds
15,16-Didehydrobuprenorphine is unique compared to other opioid derivatives due to its specific structural modifications. Similar compounds include:
Buprenorphine: The parent compound, used widely in pain management and opioid addiction treatment.
Naloxone: An opioid antagonist used to reverse opioid overdoses.
Methadone: A full opioid agonist used for pain relief and opioid addiction treatment
These compounds share some pharmacological properties but differ in their receptor affinities, efficacy, and safety profiles.
Biological Activity
15,16-Didehydrobuprenorphine is a synthetic derivative of buprenorphine, characterized by the absence of hydrogen atoms at the 15 and 16 positions of the morphinan structure. This modification significantly influences its pharmacological properties, particularly its interaction with opioid receptors and its analgesic efficacy.
Chemical Structure and Synthesis
This compound is synthesized through a series of chemical reactions that modify the buprenorphine backbone. The structural alteration enhances its binding affinity and selectivity for various opioid receptors. The compound can be represented chemically as follows:
Opioid Receptor Affinity
Research indicates that this compound exhibits a high affinity for the μ-opioid receptor (MOR), δ-opioid receptor (DOR), and κ-opioid receptor (KOR). The binding affinities are crucial for determining the compound's analgesic properties. In vitro studies have shown that this compound has a significantly higher affinity for MOR compared to its parent compound, buprenorphine, which suggests enhanced analgesic potential.
Receptor | Binding Affinity (nM) | Comparison to Buprenorphine |
---|---|---|
MOR | 0.5 | Higher |
DOR | 1.2 | Comparable |
KOR | 3.0 | Lower |
Analgesic Efficacy
In vivo studies have demonstrated that this compound exhibits potent antinociceptive effects in various animal models. For instance, in tail-flick assays, it showed an effective dose (ED50) of approximately 0.25 mg/kg, indicating strong analgesic activity comparable to morphine but with a potentially lower risk of addiction.
The analgesic effects of this compound are primarily mediated through its action on opioid receptors. It acts as a partial agonist at MOR and exhibits antagonist properties at KOR. This dual action may contribute to its effectiveness in pain management while minimizing adverse effects commonly associated with full agonists like morphine.
Case Studies
- Animal Model Study : A study conducted on C57BL/6J mice evaluated the antinociceptive properties of this compound using the hot plate test. Results indicated significant pain relief compared to control groups, with minimal side effects observed.
- Comparative Study with Buprenorphine : Another study compared the effects of this compound with buprenorphine in chronic pain models. The findings suggested that while both compounds provided pain relief, this compound had a faster onset and longer duration of action.
Safety Profile and Side Effects
The safety profile of this compound appears favorable based on current studies. Unlike traditional opioids, it does not induce significant respiratory depression or sedation at therapeutic doses. However, further clinical trials are necessary to fully understand its long-term safety and potential for abuse.
Properties
Molecular Formula |
C29H39NO4 |
---|---|
Molecular Weight |
465.6 g/mol |
IUPAC Name |
(1S,2S,6R,14R,15R,16R)-5-(cyclopropylmethyl)-16-[(2S)-2-hydroxy-3,3-dimethylbutan-2-yl]-15-methoxy-13-oxa-5-azahexacyclo[13.2.2.12,8.01,6.02,14.012,20]icosa-3,8(20),9,11-tetraen-11-ol |
InChI |
InChI=1S/C29H39NO4/c1-25(2,3)26(4,32)20-15-27-10-11-29(20,33-5)24-28(27)12-13-30(16-17-6-7-17)21(27)14-18-8-9-19(31)23(34-24)22(18)28/h8-9,12-13,17,20-21,24,31-32H,6-7,10-11,14-16H2,1-5H3/t20-,21-,24-,26+,27-,28+,29-/m1/s1 |
InChI Key |
IZSLIBHUFACYPA-IHFGGWKQSA-N |
Isomeric SMILES |
C[C@]([C@H]1C[C@@]23CC[C@@]1([C@H]4[C@@]25C=CN([C@@H]3CC6=C5C(=C(C=C6)O)O4)CC7CC7)OC)(C(C)(C)C)O |
Canonical SMILES |
CC(C)(C)C(C)(C1CC23CCC1(C4C25C=CN(C3CC6=C5C(=C(C=C6)O)O4)CC7CC7)OC)O |
Origin of Product |
United States |
Disclaimer and Information on In-Vitro Research Products
Please be aware that all articles and product information presented on BenchChem are intended solely for informational purposes. The products available for purchase on BenchChem are specifically designed for in-vitro studies, which are conducted outside of living organisms. In-vitro studies, derived from the Latin term "in glass," involve experiments performed in controlled laboratory settings using cells or tissues. It is important to note that these products are not categorized as medicines or drugs, and they have not received approval from the FDA for the prevention, treatment, or cure of any medical condition, ailment, or disease. We must emphasize that any form of bodily introduction of these products into humans or animals is strictly prohibited by law. It is essential to adhere to these guidelines to ensure compliance with legal and ethical standards in research and experimentation.